. Introduction
Despite the long period of research conducted in the Western Outer Carpathians (WOC) the genesis and tectonic evolution of their crystalline basement is still poorly recognized (e.g. Żelaźniewicz et al., 2009; Buła et al., 2015). At present the crystalline basement is hidden below thick Upper Jurassic-Neogene flysch deposits of the WOC proper (e.g. Książkiewicz, 1977; Oszczypko and Ślączka, 1985; Golonka et al., 2009). Fragments of crystalline rocks, so-called exotics (e.g. Burtanówna et al., 1937; Wieser, 1948), interpreted to be derived from uplifted ridges (cordilleras, Książkiewicz, 1953) and transported by turbidity currents and debris flows into the adjoining flysch basins, offer the opportunity to investigate the geotectonic history of these no longer existing provenance areas of the Carpathian flysch (e.g. Wieser, 1949, 1985; Książkiewicz, 1965; Oszczypko, 1975; Malik, 1978; Oszczypko et al., 2016).
A number of studies concerning the palaeogeography of the WOC have used a range of geochronological methods including K-Ar mica dating (Poprawa et al., 2004, 2005, 2006; Haber and Hałas, 2001), Th-U-total Pb monazite dating (Hanžl et al., 2000; Budzyń et al., 2008; Salata and Oszczypko, 2010; Oszczypko et al., 2016) and U-Pb zircon geochronology (Michalik et al., 2006; Poprawa et al., 2006; Budzyń et al., 2011) to constrain the timing of magmatic and metamorphic processes recorded in the exotics. The general outcome of these investigations is that numerous exclusively pre-Alpine, in parts pre-Variscan ages were determined, but no obvious correlation of stratigraphic and tectonic position and/or geography emerged. From a methodological point of view this is due to the fact that especially K-Ar mica ages are sensitive to thermal and metasomatic overprint thus obscuring any primary geochronological signal directly dating igneous or metamorphic events. This published radiometric age data set is therefore not conclusive for the unambiguous identification of protolith and metamorphic ages.
The main purpose of our study is to present and discuss zircon U-Pb ages from two exotic mega blocks (a granite from Bugaj and an orthogneiss from Andrychów), representing the alimentary crystalline basement area of the WOC flysch. Due to the size of these two exotics (> 100 m) it can safely be assumed that their loci of deposition is far more proximal to their respective shedding ridge(s) than the probably more distally derived pebbles dated in previous studies. Additionally, the latter might easily be re-sedimented making any palaeogeographic implications drawn from ages derived from such rocks severely ambiguous. The presented results provide new information to the ongoing discussion of the late Neoproterozoic palaeogeography of tectonic units which intervene between the East European Craton (Baltica) and the lithotectonic units of the Variscan orogeny (e.g. Belka et al., 2000; Nawrocki et al., 2004; Żelaźniewicz et al., 2009; Buła et al., 2015).
. Geological background
The Western Carpathian Mountains are subdivided into the Inner Carpathians, consisting of pre-Alpine crystalline basement units covered by Late Paleozoic to mid-Cretaceous sediments (e.g. Oszczypko, 2004; Poprawa and Malata, 2006) and the Outer Carpathians, separated by the narrow, strongly tectonized Pieniny Klippen Belt (Fig. 1). The WOC are depicted as a complex nappe structure consisting of Upper Jurassic to Neogene flysch deposits, that were folded and thrusted onto the pre-Alpine European foreland during the upper Oligocene to the middle Miocene (e.g. Oszczypko, 1998, 2004; Oszczypko and Oszczypko-Clowes, 2003; Golonka et al., 2005; 2009; Oszczypko et al., 2006, Ślączka et al., 2006).
Fig. 1
Simplified geological map of the Polish part of the Western Carpathians (after Żelaźniewicz el al., 2011, modified) with the location of the investigated samples (shown as yellow dots).
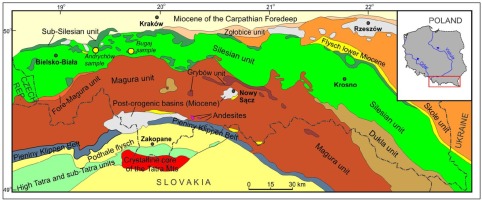
The crystalline basement constituting the pre-Alpine foreland (known only from boreholes) is divided into two basement blocks: the Upper Silesia Block (the northern part of the Brunovistulicum Terrane) to the West and the Małopolska Block to the East (Dudek, 1980; Buła, 2000; Finger et al., 2000; Żelaźniewicz et al., 2009; Buła et al., 2015). The Precambrian basement of the Upper Silesia Block is represented by a) Neoproterozoic (660–556 Ma) metamorphic and igneous rocks, b) Ediacaran anchimetamorphic flysch-type siliciclastics and c) Paleoproterozoic (2.0 Ga) metamorphic rocks with Archean (2.7 Ga) inheritance (Buła et al. 2015). The Neoproterozoic rocks of Małopolska Block are mainly represented by flysch-type series (Żelaźniewicz et al., 2009).
In the WOC several flysch basins were identified on the basis of lithostratigraphy (from south to north), i.e. the Magura, Silesian, Sub-Silesian and Skole basins (e.g. Bieda et al., 1963; Książkiewicz, 1972; Cieszkowski et al., 1985; Oszczypko, 2006). The basins were supplied with sediments from continental margins as well as from the inter-basinal elevations (ridges) separating subbasins (e.g., Książkiewicz, 1962, 1965; Unrug, 1963, 1968; Ślączka, 1986; Olszewska and Wieczorek, 2001; Poprawa et al., 2002, 2004; Golonka et al., 2008). Based on studies of palaeotransport directions it was concluded that the Brunovistulicum and/or Małopolska Terranes (external to the WOC) and the inter-basinal Silesian Ridge were the most prominent source areas of the terrigenous material (e.g. Wieser, 1949, 1985; Książkiewicz, 1965; Sikora, 1976). The Silesian Ridge developed at the boundary between Variscan and Cadomian crustal blocks of the so-called North European platform (e.g. Golonka et al., 2005; Budzyń et al., 2011). The less prominent Andrychów Ridge, recognized in the Polish WOC emerged between the Silesian and Subsilesian-Skole basins (e.g. Książkiewicz, 1960, 1977; Golonka et al., 2005) to the NNE of the Silesian Ridge. This unit is represented by several huge (> 100 m) exotic blocks, containing granitegneiss or mylonitized rocks of unknown age and Jurassic, Cretaceous and Paleogene limestones (Olszewska and Wieczorek, 2001; Ślączka et al., 2006).
. Sampling and analytical techniques
Three samples of crystalline rocks (Bu1, Bu2 and An) from exotic mega blocks (Bugaj and Andrychów) were investigated. These blocks, located on the boundary between the Silesian and Subsilesian units, are interpreted as olistoliths of the Silesian nappe (Ślączka and Kaminski, 1998; Golonka et al., 2005; Cieszkowski et al., 2009, 2012). The samples Bu1 and Bu2 were collected in the western flow of the Cedron stream in Bugaj near Kalwaria Zebrzydowska (coordinates: 49°50’56.40”N 19°40’40.70”E; Fig. 1). The sample An, representative for the metamorphic basement rocks of the Andrychów Ridge was collected from Pańska Góra Hill, SE of Andrychów (coordinates: 49°51’10.17”N 19°21’28.95”E, Fig. 1). The two samples Bu1 and An, weighting about 25 kg each, were selected for U-Pb dating.
Whole-rock and mineral analysis
Whole-rock samples were analysed by X-ray fluorescence (XRF) for major and large ion lithophile trace Elements (LILE) and by fusion and ICP-MS for high field strength elements (HFSE) and rare earth elements (REE) in the Bureau Veritas Minerals (Canada). Preparation involved lithium borate fusion and dilute digestions for XRF and lithium borate decomposition or aqua regia digestion for ICP-MS. LOI was determined at 1000°C. REE were normalized to C1 chondrite (Sun and McDonough, 1989).
Microprobe analyses of rock-forming and accessory minerals were undertaken in the Inter-Institutional Laboratory of Microanalyses of Minerals and Synthetic Substances, Warsaw, using a Cameca SX 100 electron microprobe operating in the wavelength-dispersive spectroscopic (WDS) mode with the following conditions: 15 kV accelerating voltage, 20 nA beam current, 1–5 μm beam diameter, peak count-time of 20 s and background count-time of 10 s.
Rb-Sr and Sm-Nd whole rock isotope analysis
The Rb-Sr and Sm-Nd analytical work was performed at the Laboratory of Geochronology, Department of Lithospheric Research, University of Vienna. Rb, Sr, Sm and Nd concentrations were determined from two different sample aliquots by isotope dilution (ID) using 87Rb-84Sr and 147Sm-150Nd spikes. Rb, Sm and Nd ID samples were measured as metals from a Re (single: Rb; double: Nd and Sm) filament using a Finnigan™ MAT262 mass spectrometer, while Sr ID and isotope composition (IC) and Nd IC samples were run on a ThermoFinnigan™ Triton TI TIMS machine. Maximum total procedural blanks were < 1 ng for Sr and 50 pg for Nd and were taken as negligible. Within-run mass fractionation for Nd and Sr isotope compositions (IC) was corrected to 146Nd/144Nd = 0.7219, and 86Sr/88Sr = 0.1194, respectively. Uncertainties on the Nd and Sr isotope ratios are quoted typical as 2σm. Errors for the 87Rb/86Sr and 147Sm/144Nd ratios, respectively, are taken as ±1%, or smaller, based on iterative sample analysis and spike recalibration. During the period of investigation a 143Nd/144Nd ratio of 0.511850 ± 0.000005 (n = 5) and a 87Sr/86Sr ratio of 0.710274 ± 0.000008 (n = 5) were determined for the La Jolla (Nd) and the NBS987 (Sr) international standards, respectively. Details of techniques, accuracy and data precision of Rb-Sr and Sm-Nd isotopic measurements are given by Thöni et al. (2008).
Zircon separation, CL imaging and U-Th-Pb MC-ICP-MS dating
Zircon crystals were separated using standard techniques (crushing, hydrofracturing, washing, Wilfley table, magnetic separator and handpicking). The separation was carried out in the Institute of Geological Sciences, Polish Academy of Sciences, Cracow. Zircon grains were selected for the morphological study using scanning electron microscopy and then imaged by cathodoluminescence using a FET Philips 30 electron microscope (15 kV and 1 nA) at the Faculty of Earth Sciences, University of Silesia, Sosnowiec, Poland.
The LA-MC-ICP-MS (Laser Ablation Multi Collector ICP-MS) analytical work was performed in two sessions at the joint ICP-MS laboratory of the Department of Earth Sciences, Karl-Franzens-University Graz and the Institute of Applied Geosciences, Graz Technical University. Analytical procedures followed the methodology outlined in Klötzli et al. (2009). Zircon 206Pb/238U, 207Pb/206Pb, and 208Pb/232Th ratios and ages were determined using a 193nm Ar-F excimer laser (NewWave) coupled to a multi-collector ICP-MS (Nu Instruments Plasma II). Ablation using He as carrier gas was raster-wise according to the CL zonation pattern of the zircons. Line widths for rastering were 10–15 μm with a rastering speed of 5 μm/sec. Energy densities were 5–5.5 J/cm2 with a repetition rate of 10 Hz. The He carrier gas (0.7 l/min) was mixed with the Ar carrier gas flow prior to the ICP plasma torch. Ablation duration was 30 to 70 sec with a 30 sec gas and Hg blank measurement preceding ablation. Ablation count rates were corrected accordingly using the mean blank measurement intensities of the individual masses. Remaining counts on mass 204 were interpreted as representing 204Pb. Static mass spectrometer analysis was as follows: 238U and 232Th were measured in Faraday detectors, 208Pb, 207Pb, 206Pb, 204Pb+204Hg and 202Hg in discrete ion counter detectors. An integration time of 1 sec was used for all measurements. The ion counter - Faraday and inter-ion counter gain factors were determined before the analytical session using the reference zircon Plešovice (Sláma et al., 2008). The overall sensitivity for 206Pb on reference zircon Plešovice was c. 0.18 mV/weight-ppm 206Pb. For 238U the corresponding value was c. 0.39 mV/weight-ppm U. Isotopic ratios were calculated using the MS Excel spreadsheet LamTool U-Th-Pb version VI (Košler et al., 2008; Košler and Klötzli, unpublished). In order to avoid potential surface contamination, the signals from the first laser pass was rejected and untypical Pb-peaks, from Pb-rich inclusions, were removed in the LamTool spreadsheet. Mass and elemental bias and mass spectrometer drift of U/Pb, Th/Pb and Pb/Pb ratios, respectively, were corrected applying the “intercept method” of Sylvester and Ghaderi (1997). The calculated 206Pb/238U and 207Pb/206Pb intercept values, respectively, were corrected for mass discrimination from analyses of reference zircon Plešovice zircon (Sláma et al., 2008) measured during the analytical session using a standard bracketing method (Klötzli et al., 2009). The correction utilizes regression of standard measurements by a quadratic function. No common Pb correction was applied to the data. Final age calculations were performed with Isoplot© 3.75 (Ludwig, 2012). Reference zircon Plešovice (Sláma et al., 2008) was also used as secondary standard in order to derive an estimate of the overall uncertainties associated with the age determinations. 4 and 7 measurements where additionally made during the two analytical session, which resulted in concordia ages of 337.2 ± 3.6 Ma and 337 ± 10 Ma, respectively. These are within error identical to the accepted reference 206Pb/238U age of 337.13 ± 0.37 Ma (Sláma et al., 2008). These uncertainties where propagated in quadrature to the single dates in order to account for the overall analytical dispersion of the LA method (Klötzli et al., 2009). All errors reported for LA data are at the 2-sigma level.
. Petrography and whole-rock geochemistry
Bugaj granite mega block
The samples collected from the Bugaj mega block are coarse-grained granite (Bu1, Fig. 2a, Fig. 3) to granodiorite (Bu2, Fig. 3), lacking any visible orientation, locally porphyritic. They are composed of pinkish K-feldspars (Or91-93Ab5-6Cn1-2), weakly zoned oligoclase (An18-25), biotite showing a brown-yellow pleochroism (Ti = 0.38– 0.42 a.p.f.u.; #fm = 0.65–0.66), locally replaced by chlorite and associated with secondary rutile. Accessory minerals are (Ca, F)-apatite and zircon. Sporadically rounded monzonitic enclaves up to 5–10 cm in size can be found, showing a sharp contact with the surrounding host rock. The enclaves are composed of fine-grained biotite (Ti = 0.30–0.38 a.p.f.u.), oligoclase (An20-26), and quartz. Accessory (Ca, F)-apatite is also observed (BuC, Fig. 3).
Fig. 2
Field photo (a) of the Bugaj granitoid (sample Bu1) and field photo (b) of the Andrychów orthogneiss (sample An).
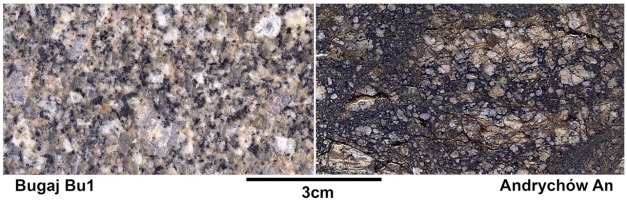
Fig. 3
Classification position of the Bugaj granitoids in: (a) alkali (K2O + Na2O) versus SiO2 (TAS) classification diagram (Middlemost, 1985); (b) Primitive mantle-normalized (Sun and McDonough, 1989) multielement “spider” diagrams; (c) classification position of the Bugaj granitoids in Multicationic R1–R2 diagram (De La Roche et al.,1980), with fields numbered according to Batchelor and Bowden (1985); 1 – mantle fractionates, 2 – pre-plate collision suites, 3 – post-collision suites, 4 – late orogenic magmas, 5 – anorogenic suites, 6 – syncollisional (anatectic) suites. R1 = 4Si − 11(Na + K) − 2(Fe + Ti); R2 = 6Ca + 2 Mg + Al.
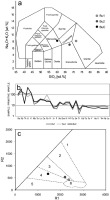
Studied rocks are peraluminous (ASI = 1.16–1.23) with silica contents around 60–71 wt.%, K2O ≥ Na2O and Rb/Sr ratios = 1.1–1.4 (Table 1). The rocks belong to the high K-calc-alkaline series and are intermediate between the ferroan and magnesian families of the Frost and Frost (2008) geochemical classification. Chondrite-normalized (Sun and McDonough, 1989) REE patterns show low LREE enrichment (CeN/YbN = 3.18–6.34) and a strong negative Eu anomaly (Eu/Eu* = 0.38–0.57; Table 1; Fig. 3b). Magma crystallization temperatures calculated on the basis of the Zr-saturation geothermometer of Watson and Harrison (1983) are in the range of 800–850°C
(Table 1). The primitive-mantle-normalized multielement diagram points to an arc-setting of the magma, with typical Nb and Ta negative anomalies, whereas the Batchelor and Bowden (1985) classification suggests a post-collisional to late-orogenic magmatic suite (Fig. 3b, 3c). Granodiorite-granite rocks show relatively high
Table 1
Chemical composition and selected petrological indicators of Bugaj granitoid rocks.
[i] Explanations
LoD – limits of detection; LOI – loss of ignition; Eu/Eu* = Eu/√Sm·Gd; ASI = Al2O3/(CaO + Na2O + K2O − 3.33 P2O5) (in molecular values); TZr = temperature calculated according to Watson and Harrison (1983) procedure.
ThN/UN ratios (2.06 and 1.05 respectively), whereas the monzonitic enclave shows a strong positive U anomaly and a ThN/UN ratio of 0.03, suggesting a highly oxidised source (possibly mantle-type?) of the monzonitic magma (Fig. 3b; Table 1).
The whole-rock isotope geochemistry data of two Bugaj mega block samples are presented in Table 2 Both samples (Bu1, Bu2) show nearly identical 87Rb/86Sr ratios (3.154 ± 0.032 and 3.236 ± 0.032) as well as 87Sr/86Sr ratios (0.756053 ± 0.000004 and 0.755499 ± 0.000004). The initial 87Sr/86Sr(580 Ma) ratios are 0.72997 and 0.72874.
Table 2
Rb-Sr and Sm-Nd whole rock isotope analysis of Bugaj granitoid rocks.
Contrary to the above the Sm-Nd isotope systematics of the two Bugaj mega block samples are dissimilar: 147Sm/144Nd ratios are 0.1521 ± 0.0015 (Bu1) and 0.1366 ± 0.0014 (Bu2). The 143Nd/144Nd ratios are 0.512397 ± 0.000004 and 0.512428 ± 0.000005, respectively. The resulting εNd580 are –1.4 and 0.4.
Andrychów orthogneiss mega block
The sample (An) is a medium-grained augen gneiss (Fig. 2b), consisting of quartz, K-feldspar, plagioclase (An10-18) and biotite. The foliation is defined by biotite, quartz ribbons and elongate feldspars. Accessory minerals are apatite and zircon. Due to the penetrative low-temperature and structural overprinting of the Andrychów mega block rocks, no detailed micro-chemical analyses, whole rock chemistry as well as Rb-Sr and Sm-Nd isotope whole rock geochemistry were obtained.
. Zircon characteristics and U-Pb ages
Bugaj mega block
The zircons are colourless or slightly pink, euhedral, normal to very long-prismatic, even to acicular (aspect ratios 1:1 to 1:10). Grain sizes vary in length from ca. 50 to 350 μm (Fig. 4). A small percentage of zircon grains show an unusual habit contrasting markedly with the prismatic and acicular crystals (Fig. 4, p6Bu81). This habit is tabular, strongly flattened on the [110] crystal face (elongation ratio < 1). The typology falls into the S4 subtype group of Pupin (1980).
Fig. 4
Secondary electron (SE) images of selected zircon crystals from granite sample Bu1. See text for description.
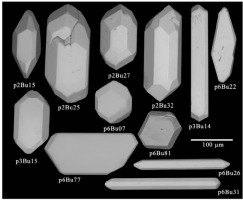
The cathodoluminescence (CL) images reveal a complexity of the internal structure. A significant number of crystals shows igneous oscillatory growth zoning, with growth bands varying between fine and broad within individual grains (Fig. 5). Luminescence is variable, but mostly moderate. Some grains display a blurred, light grey zonation, present in the centre of the zircon crystals (Fig. 5, p3Bu01). A small number of inherited cores were observed in normal-prismatic crystals (Fig. 5).
Fig. 5
Compiled cathodoluminescence (CL) images showing the range of textures observed in zircon crystals from granite sample Bu1. See text for details. The white rectangles show the approximate location of laser ablation trenches (confirmed by re-inspecting grains under CL after the LA analysis) and are not to scale. The numbers refer to the analytical data presented in Table 3.
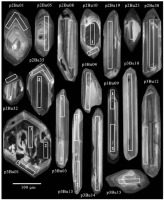
Table 3
LA-MC-ICP-MS U–Pb zircon data from exotic mega blocks (samples An and Bu1).
Crystal domains targeted for dating are characterized by the presence of a well-developed fine-scale oscillatory growth zonation (Fig. 5). 25 dates on 19 crystals were gained (Table 3, Fig. 5). Individual 206Pb/238U dates range from 1751 ± 78 Ma to 431 ± 19 Ma, whereas 207Pb/206Pb dates range from 1773 ± 25 Ma to 510 ± 9 Ma, respectively. No systematic difference between the two populations could be detected. Thus the final age calculation took into account all analysed crystals of the Bugaj1 sample. One analysis (Bugaj1_p2bu_023A) is concordant at an age of ca. 1760 Ma (Fig. 6a). 14 dates form a sub-concordant (discordance < 5%) cluster with a lower intercept date of 580.1 ± 6.0 Ma (Fig. 6b, model 1 solution, anchored at 207Pb/206Pb = 0.85 ± 0.05, MSWD = 1.8, Probability of fit = 0.031). These crystals show a mean Th/U of 0.16 ± 0.07. Three sub-concordant analyses (discordance < 5%), characterized by comparably high Th/U (0.30 ± 0.04), form a cluster with a lower intercept date of 662 ± 37 Ma (not shown, model 1 solution, anchored at 207Pb/206Pb = 0.85 ± 0.05, MSWD = 0.12, Probability of fit = 0.89). This lower intercept date is statistically distinguishable from the former one, also based on the disparate Th/U ratios of the two clusters. The remaining 7 analyses are highly discordant with high Th/U ratios (0.72 ± 0.35), again statistically distinguishable from the former two clusters.
Andrychów mega block
Zircon grains are euhedral, up to 300 μm long, with aspect ratios from 1:1 to 1:3 (Fig. 7). They are clear, yellow and greenish yellow in colour.
In CL images a variably developed oscillatory growth zoning is the prominent feature of all analysed grains, as shown by moderate to weak luminescence. Some crystals display core-rim structures. The cores are characterized by oscillatory growth zoning with high/moderate CL intensity (Fig. 8).
Fig. 7
Secondary electron (SE) images of selected zircon crystals from orthogneiss sample An. See text for description.
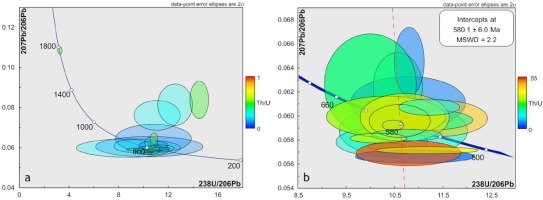
Fig. 8
Compiled cathodoluminescence (CL) images showing the range of textures observed in zircon crystals from orthogneiss sample An. See text for details. The white rectangles show the approximate location of laser ablation trenches (confirmed by re-inspecting grains under CL after the LA analysis) and are not to scale. The numbers refer to the analytical data presented in Table 3.
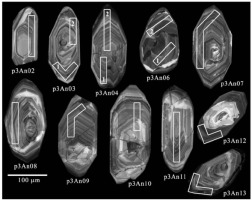
Crystal domains targeted for dating are characterized by the presence of a well-developed fine-scale oscillatory growth zonation (Fig. 8). 15 dates on 10 crystals were gained (Table 3, Fig. 8). Individual 206Pb/238U dates range from 2149 ± 284 Ma to 319 ± 73 Ma, whereas 207Pb/206Pb dates range from 2324 ± 295 Ma to 554 ± 29 Ma, respectively. One analysis (Andrychów_p3an_06A) is concordant at an age of 2123 ± 23 Ma (Fig. 9a). 7 dates form a sub-concordant (discordance < 5%) cluster with a mean Th/U of 0.23 ± 0.11. Two lower intercept dates can be calculated: i) 549 ± 29 Ma with an upper intercept at 2432 ± 1500 Ma (not shown, model 1 solution, MSWD = 0.99, Probability of fit = 0.42); ii) 542 ± 21 Ma with the upper intercept fixed at 2123 ± 23 Ma of analysis Andrychów_p3an_06A (Fig. 9b, model 1 solution, MSWD = 0.86, Probability of fit = 0.53).
Fig. 9
Tera-Wasserburg diagrams for isotopic ratio for zircon crystals from orthogneiss sample An: a) all data points; b) concordant to sub-concordant data points defining a discordia line with a lower intercept of 542 ± 21 Ma (forced through data point p3An_06/1). The inherited xenocrysts (p3An_06/1, p3An_06/2) and the two data points showing recent lead loss (p3An_08, p3An_13) are not shown.
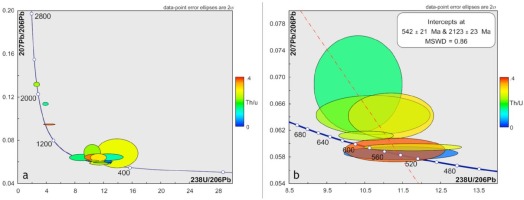
The calculated upper intercept value of 2432 ± 1500 Ma of i) is well within uncertainty with the concordant age value of 2123 ± 23 Ma used for the calculation in ii). Also the two lower intercept ages are identical within their uncertainty limits.
The remaining analyses either are strongly discordant (discordance > 10%) or sub-concordant (discordance < 5%) at 207Pb/206Pb ages between 2324 ± 295 Ma and 624 ± 29 Ma. The Th/U ratios of these analyses are identical to the former one (0.18 ± 0.06).
. Discussion
Exotics represent one of the most important sources of information about Carpathian alimentary areas, indirectly indicating their geotectonic history. For both exotic mega blocks (sample Bugaj and sample Andrychów) igneous formation ages were derived from the zircon U-Pb data.
The Cadomian age (580.1 ± 6.0 Ma) from sample Bu1 is interpreted as magmatic crystallization age of the Bugaj granitoids. A fine-scale oscillatory zonation in the CL images as well as Th/U ratio of 0.16 ± 0.07 point to igneous crystal growth (Corfu et al., 2003). Three analyses (Th/U ratios 0.30 ± 0.04), forming a cluster with a lower intercept age of 662 ± 37 Ma are interpreted to reflect a major contribution from a single inheritance source to the ca. 580 Ma igneous event. The singular 207Pb/206Pb age of 1773 ± 25 Ma (p2Bu_23) represents a xenocryst.
The Sr and Nd whole-rock isotope data are controversial. The initial (at ca. 580 Ma) 87Sr/86Sr ratios of the Bugaj granitoids (0.72997 and 0.72874) are highly radiogenic, pointing to the assimilation of an older, possibly strongly Rb enriched source to the Bugaj melt. On the other hand, the Nd isotope systematics (εNd580 –1.4 and 0.4) rather point to a significant contribution of a distinct mantle source to the Bugaj melt. These geochemical data thus allow to recognize a hybrid geochemical character of the investigated rocks and/or decoupling of the Rb-Sr and Sm-Nd isotopic systems during post-magmatic alteration also supported by the presence of mafic enclaves. Such a hybrid character is also reflected in the high K-calcalkaline series affinity with the intermediate association of the ferroan and magnesian families (Frost and Frost, 2008). Also the relatively high ThN/UN ratios (2.06 and 1.05) are remarkably well reflected in the high Th/U ratios of some zircon domains (0.72 ± 0.35). It is not clear, whether this hybridisation is a primary one, i.e. occurring during protolith formation or a secondary one.
The biotite K-Ar age of 485 ± 10 Ma (Haber and Hałas, 2001) indicates either slow cooling to ca. 300°C after the 580 Ma magmatic event or a Lower Ordovician thermal and possibly fluid-related overprint, which might have caused the resetting of the Rb-Sr system which resulted in highly radiogenic 87Sr/86Sr ratios. It has to be noticed however, that such an event is not detected in the U-Pb zircon data. This fact is in favour of the slow cooling model.
In the Andrychów sample, the lower intercept age of 542 ± 21 Ma, obtained from zircon domains exhibiting a fine-scale oscillatory growth zonation in the CL images, is interpreted as the uppermost Proterozoic to Cambrian magmatic crystallization age of the granitic precursor of the orthogneiss. The concordant age at 2123 ± 23 Ma represents Palaeoproterozoic inheritance. There is no evidence for a Variscan (Devonian-Carboniferous) or Alpine (Neogene) metamorphic overprint, neither from the zircon U-Pb age systematics nor from the zircon mineral chemistry, i.e. the Th/U ratios (samples Bu1 and An), and the whole-rock isotope geochemistry (sample Bu1). Lack of any Variscan overprint suggests no similarities of the analysed exotic mega blocks to the crystalline basement of the Inner Western Carpathians, showing the Variscan consolidation, e.g. from granitoids of the Tatra Massif (e.g. Burda and Klötzli, 2011; Burda et al., 2013).
The U-Pb zircon ages presented in this study are consistent with published results (monazite Th-U-total Pb dating, K-Ar dating of muscovite and biotite), reported from clastic material supplying Silesian basin from the Brunovistulicum and/or Małopolska Terranes as northern source areas (Poprawa et al., 2004, 2005, 2006; Budzyń et al., 2008, 2011).
The sequence of events obtained from U-Pb zircon ages from Bugaj and Andrychów exotic mega blocks shows similarities to magmatic-metamorphic events identified in the Brunovistulicum Terrane. These events include: a) terrane collision with deformation, metamorphism and plutonism at ca. 650–620 Ma; b) arc-type granitoid intrusions at ca. 590–580 Ma and c) late bimodal magmatism and strike-slip deformation at ca. 560–550 Ma. No evidence for Variscan magmatism and/or metamorphism has emerged (e.g. Finger et al., 2000; Żelaźniewicz et al., 2009 and references therein). Also the εNd580 (–1.4 and 0.4) obtained for the Bugaj granitoid is similar to the Neoproterozoic igneous rocks from the Brunovistulicum Terrane having εNd580 values ranging from –1 to +3 (Finger et al., 2000).
Our investigations thus support the connection of the exotic mega blocks with the Avalonian-Cadomian orogenic belt during the Neoproterozoic (Żelaźniewicz et al., 2009).
. Conclusions
For both exotic mega blocks (Bugaj and Andrychów) igneous formation ages could be derived from the zircon U-Pb data. These are 542 ± 21 Ma for the orthogneiss from Andrychów and 580.1 ± 6.0 Ma for the granitoid from Bugaj.
The U-Pb zircon ages, derived from > 100 m sized exotic mega blocks, directly reflect the presence of substantial amounts of a proximal ‘Cadomian’ aged crust proper in the vicinity of the WOC basement. This is a marked difference to earlier published data, which are derived from cm-dm sized pebbles only. As these can easily be derived from re-sedimented sources the ages do not necessarily reflect direct derivation from a ‘Cadomian’ basement, inasmuch as transport distances are not known.
Exotic mega blocks deposited to the WOC basins were related to the Brunovistulicum Terrane. They belong to the group of Vendian/Early Cambrian granitoids, representing the latest, post-tectonic expression of the Cadomian cycle.