. Introduction
Cathodoluminescence (CL) is a visible light of emissions when a material is irradiated by an electron beam. The luminescence phenomenon mainly occurs due to a presence of specific luminescence center due to lattice defect or impurity. The CL features in minerals are closely related to varieties of emission centers such as the impurity concentration, defect density, crystal fields (e.g., Marfunin, 1979). Therefore, a CL method has a high advantage to reveal internal textures, growth zoning and antigenic minerals, which are difficult to identify by conventional optical examinations such as a polarization microscopy (e.g., Matsunami et al., 1993). Recently, CL microscopy and spectroscopy have been extensively used for planetary sciences to characterize extraterrestrial minerals, most of which were formed under unusual conditions such as shock metamorphism, high-temperature melting and super-reductive environment (e.g., Gucsik, 2009). A CL color imaging was employed to evaluate a petrologic type of enstatite chondrite (E-chondrite) formed in a reducing environment (e.g., Zhang et al., 1996), which is divided into two groups of EL and EH chondrite by remaining chondrule size and Fe-Ni-metal abundance. The EH chondrite exhibits a brighter blue CL in the enstatite at higher petrologic type, whereas the EL chondrite has the enstatite at higher petrologic type with a brighter magenta CL.
Enstatite is one of most important rock-forming minerals in the terrestrial and extraterrestrial materials. The enstatite CL in the meteorites has been investigated to reveal their thermal histories (e.g., Derham et al., 1964; Keil, 1968; Zhang et al., 1996). Most of enstatite which emits various CL has been found in E-chondrite and enstatite achondrite (Aubrite). Major silicate phase in E-chondrite and Aubrite is a near endmember of enstatite (FeO <1.0%). Minute amounts of quencher ions such as Fe2+ ion by the segregation of metal iron under a reductive condition (e.g., Keil, 1968, Weisberg et al., 1994) result in various CL emissions with red, purple and blue (Reid et al., 1964).
Yamato 86004 (hereafter Y-86004) is one of Antarctic E-chondrite classified as an EH melt rock, which is characterized by constituent minerals having a feature of crystallization from melts with an Abee matrix (Rubin and Scott, 1997). We have found a CL zonation composed the enstatite with various color in Y-86004 for the first time from the meteorites. In this study, we have conducted to clarify the CL zonation in Y-86004 using CL color imaging and spectroscopy for estimating a thermal history of the meteorite.
. Sample and Methods
The sample of Y-86004 was provided as a polished thin section by the National Institute of Polar Research (NIPR), Japan. It is a rounded shape with ~4.5 mm in diameter (Fig. 1b). Y-86004 was identified as an E6 chondrite by Yanai and Kojima (1995). Subsequently, it has been classified into a EH melt rock judging by petrographical and mineralogical characteristics (Lin and Kimura, 1998).
Figure 1
(a) Color CL image of Y-86004. Yellow cross marks indicating the positions in CL-spectral measurement. (b) Parallel-polarizing microscope image of Y-86004 corresponding to the area in Fig. 1a. (c) Color-CL image of Y-86004 indicating boundaries between each zone. Zone 1: blue-CL area, zone 2: light-blue-CL area, zone 3: red-CL area. (d) BSE image of Y-86004 corresponding to the area indicating a square in Fig. 1c. Zone 1: blue-CL area, zone 2: light-blue CL area, zone 3: red-CL area, zone 4: non-CL area (fusion crust). en: enstatite, tri: tridymite, pl: plagioclase and opq: opaque minerals.
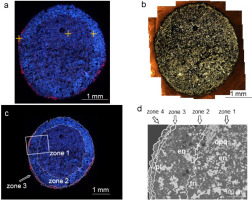
CL color images were obtained using the Luminoscope (ELM-3), attached with a cooled charge-coupled device (CCD) camera, which consists of a cold cathode discharge tube and a vacuum chamber in which the sample is placed. It was operated stably with electron beams generated by an excitation voltage of 15 kV and a beam current of 0.5 mA. CL spectroscopy was carried out by an SEM-CL system, which consists of an SEM (JEOL, JSM-5410LV) combined with a grating monochromater (Oxford, Mono CL2) with operating conditions of 15 kV and 1.0 nA in a scanning mode. The CL emitted from the sample was collected in the range of 300–800 nm with a photomultiplier tube by a photon counting method. All CL spectra were corrected for total instrumental response, which was measured using of a calibrated standard lamp (Eppley Laboratory: Quartz Halogen Lamp). This correction prevents errors in the peak position of emission bands and allows quantitative evaluation of CL intensity. Detailed construction of the equipment and the analytical procedure can be found in Ikenaga et al. (2000). Crystal–chemical characterization of the constituent minerals was performed using the JXA-8230 electron probe microanalyzer (EPMA) and Nicolet Almega-XR Raman spectrometer.
. Results and Discussion
Y-86004 shows a rounded shape of ~4.5 mm diameter in a thin section, which is completely surrounded by a fusion crust. It exhibits no texture with chondrules and chondrule fragments under a polarizing microscope. According to EPMA and Raman spectroscopy analyses, the meteorite contains orthopyroxine of near-end-member enstatite (20–200 μm), opaque minerals of metallic Fe-Ni and troilite (up to 200 μm), plagioclase with albite composition (up to 30 μm) and silica mineral of tridymite (up to 120 μm) and glassy materials mostly in the fusion crust. Orthoenstatite (Oen) is predominant phase, which occurs as euhedral lath-shape grains, which usually protrude into or enclosed in opaque minerals. Plagioclase and silica mineral are commonly found as interstitial materials in the Oen grains. The petrographic texture characterized by enstatite occurrences in Y-86004 is similar to that observed in the EH melt rock, which may have experienced an impact melt near the surface of the parent body (Rubin and Scott, 1997; Lin and Kimura, 1998). However, Y-86004 has no impact-shock textures (e.g., planar fractures, clinoenstatite lamellae, and mosaicism) in the constituent minerals.
Chemical compositions of the enstatite with different CL color are compiled on an average of six analyses per grain by EPMA (WDS) in Table 1, additionally with glassy material in the fusion crust. Each enstatite is a nearly end member with low FeO contents (<1 wt.%), whereas glassy material has a similar composition pyroxene (diopside) with high FeO contains (> 30 wt.%).
Table 1
Chemical compositions on an average of three analyses of the enstatite with different CL colors and fusion crust in each zone. n.d.: not detected.
CL observation
Y-86004 shows a rounded shape with blue luminescence as the whole pattern in a color CL image (Figs. 1a and 1c), whereas it has a thin red rim. According to Zhang et al. (1996), the EH chondrite with a higher petrographic type (e.g., EH5 chondrite) exhibits more predominant blue CL in contrast with red CL for that with a low petrographic type (e.g., EH3 chondrite). Therefore, Y-86004 should be corresponding to E-chondrite judging from its CL color.
The enstatite with low FeO contents (<5 wt.%) in E-chondrites shows visible CL due to a negligibly small concentration of quencher (e.g., Keil, 1968; Weisberg et al., 1994), which is mostly Fe2+ ion. Extrinsic luminescence of enstatite should be controlled mainly by the concentrations of activators (Mn2+ and Cr3+ ions) and quencher (Fe2+ ion).
Color CL imaging in the thin section reveals a concentric zonation mainly attributable to the distribution of the enstatite different in color as arranged from within outward blue CL (zone 1), light blue CL (zone 2), red CL (zone 3) and non-CL zone (zone 4) corresponding to a fusion crust (Figs. 1a and 1c). BSE image (Fig. 1d) shows none of concentric features as found in a color CL image, neither in the petrographic examination under a polarizing microscope (Fig. 1b). Concentric CL zoning has been found in the meteorites for the first time, although a concentric CL image was observed only in the chondrule (forsterite) in Semarkona chondrite (Matsunami et al., 1993).
Average width of each band in the zonation is ~1.50 mm for zone 1, ~700 μm for zone 2, ~100 μm for zone 3 and ~80 μm for zone 4. Zone 1 and 2 consist of blue and light blue enstatite, dark blue tridymite, reddish-brown plagioclase, and non-CL grains of opaque minerals. These zones have similar petrographic texture, however, the distribution ratio of light-blue-CL enstatite in zone 2 is higher than that of zone 1. Zone 3 is characterized by red-CL enstatite with opaque minerals, but no tridymite. Zone 4 composed of glassy materials including blebs and opaque minerals exhibits no emission, suggesting heating above the melting temperature of the enstatite. Therefore, color CL zonation consisted of the enstatite with various colors should record a thermal history of the meteorite in the process of rapid heating and quenching during a flash heating when it entered into the atmosphere.
CL spectroscopy and spectral deconvolution
The CL spectra of the enstatite in each zone are shown in Fig. 2. All of them have two broad emission bands at around 400 nm in a blue region and at around 670 nm in a red region. Spectral deconvolution of the CL using a Gaussian curve fitting were carried out to clarify the emission components derived from various emission centers by the method proposed by Ohgo et al. (2015). The results of the spectral deconvolution reveal five emission components in their spectra (Figs. 3, 4, and 5). The component at 1.71 eV is assigned to an impurity center activated by Cr3+ ion substituted for Mg in M1 site as an electron transition of 4T2 → 4A2. The component at 1.86–1.91 eV is ascribed to an impurity center of Mn2+ ion substituted for Mg in M2 site as an electron transition of 4T1g → 6A1g. Both of Cr3+ and Mn2+ centers have been recognized in extraterrestrial and synthetic enstatite (Steele, 1988; Moncorgé et al., 1999; Catalano et al., 2014).
Figure 3
Deconvolution of CL spectrum of blue-CL enstatite in energy units by Gaussian curve fitting.
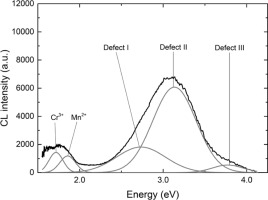
Figure 4
Deconvolution of CL spectrum of light-blue-CL enstatite in energy units by Gaussian curve fitting.
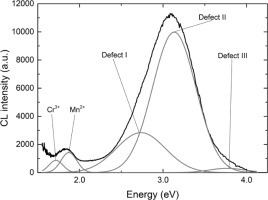
According to Ohgo et al. (2015), the component at 2.73 eV can be assigned to an emission center (Defect I) related to ‘intrinsic defect’ formed during crystal growth. Such defect center has been detected in synthetic enstatite without any activator and quencher ions (e.g., Lofgren and Dehart, 1992). An analogous intrinsic defect center also has been found at 2.75 eV in synthetic forsterite, which is one of common Mg-silicate minerals associated with enstatite in meteorites. Furthermore, forsterite has emission components of Cr3+ ion at 1.65–1.72 eV, Mn2+ ion at 1.92 eV, defect center at 2.75 and 3.15 eV (Gucsik et al., 2012), all of which accord closely with corresponding components obtained from the CL spectra of the enstatite in zone 1, 2 and 3. Ohgo et al., (2015) presumed that the emission component at 3.13–3.15 eV might be due to the defect center (Defect II) related to structural distortion of T(Si, Al)-O chain when Al substitutes for Si and/or deformation of the lattice due to an incorporation of Ca and Ti ions. In the case of measured enstatite, however, too low concentrations of Ca and Ti makes more difficult to judge the effect of impurity elements on the lattice in the enstatite (Table 1). According to Ohgo et al. (2015), the emission component at 3.77 eV in zone 1–3 enstatite might be related to the defect center (Defect III) attributable to cosmic-ray irradiation on the surface of the parent body and during traveling in space. Cosmic rays with high energy could flick out oxygen in enstatite crystal structure and form oxygen vacancy related to defect center with a near-UV emission.
Formation of CL zonation
Color CL image (Fig. 1) shows four CL zones arranged in a concentric pattern. As demonstrated by Zhang et al. (1996), the CL of the enstatite in E-chondrite reflects a petrographic type and a peak temperature during thermal metamorphism in space. Therefore, thermal history of Y-86004 might be recorded as its CL zonation. Y-86004 experienced a melting on the surface of a parent body possibly related to a heavy shock event (Lin and Kimura, 1998) and a rapid cooling from the melt. It is supported by the presence of tridymite, which emerges by a quenching from high temperature (867 < T < 1470°C) (Swamy et al., 1994; Kimura et al., 2005). Therefore, all of the enstatite in Y-86004 were formed in a quenching process near surface of the parent body, suggesting that original enstatite in Y-86004 should be Oen with a blue CL emission.
The result of polarization microscopy and Raman spectroscopy show two types of enstatite with blue and light-blue CL in zone 2, former of which is virtually identical to Oen with a blue emission in zone 1. The light-blue enstatite has two defect centers of Defect I and Defect II, whereas the enstatite is identified as Oen. It is characterized by the Defect II not detected in the blue enstatite. Inasmuch as the meteorite experienced a flash heating above the melting point of the enstatite, the heating and cooling process during a falling through the atmosphere might affect a creation of defect center (Defect II) and alteration of existing defect (Defect I). Therefore, according to Gasparik (1990), some portion of the enstatite in zone 2 probably might have experienced a phase transition from Oen to protoenstatite (Pen) during a flash heating, which occurs at near 1273 K. In this case, the enstatite with blue CL considerably survived from the original enstatite the same to one in zone 1, suggesting the temperature at around 1273 K. When Pen is rapidly cooled, the phase transition involves physical stress, which might create defect center (Defect II) in the structure.
The enstatite in zone 3 is identified as LT-Cen by a Raman spectroscopy. This result is also supported by the peak energy of the emission component related to Mn2+ impurity. According to Ohgo et al. (2015), CL feature of red emission is useful to distinguish between Oen and LT-Cen phases. In the terrestrial enstatite, Oen has an emission component of impurity Mn2+ ion at 1.85 eV, but at 1.90 eV for LT-Cen. The enstatite in zone 3 has a corresponding component at 1.92 eV, suggesting identification of LT-Cen. According to Gasparik (1990), when the Oen experienced high temperature at a temperature near the melting point of enstatite, it transfers from Oen and Pen to HT-Cen, which is stable near the melting point at 1831 K. Therefore, red-CL enstatite in zone 3 might be formed by a rapid quenching from HT-Cen at around 1831 K due to structural similarity between LT-Cen and HT-Cen. The enstatite in zone 3 has almost none of blue emission related to the defects centers, implying the elimination of lattice defect by the elevated temperature near the melting point without structural stress in the transition between HT-Cen and Oen.
Y-86004 suffered a heavy ablation on its surface, which took away melting materials from the meteorite body in a short period. Zone 4 was formed during such ablation with amorphousization of enstatite-rich materials at the surface of the meteorite. Therefore, the surface of the meteorite has been exposed at and above the temperature of a melting point (>1831 K) of the enstatite.
Y-86004 was abruptly heated for a short time when it entered the earth's atmosphere, and rapidly quenched in the Antarctic ice immediately after its falling. In this study, the enstatite with a background of previous phases corresponding to the elevated temperatures can be characterized by CL imaging and spectroscopy, which reveals a mechanism of CL color zonation found in Y-86004.
Estimation of thermal distribution in the meteorite
We have conducted to evaluate the temperate history of Y-86004 during heating when the meteorite entered into the atmosphere. In general, typical falling object collides with the Earth’s atmosphere at velocities of 12–20 km·s–1 (Bottke et al., 1994), where the most likely impact angle is 45° (Shoemaker, 1962). According to Sears (1975), the time to flash heating of meteorite (luminous flight time) is a few tens of seconds. When thermal conductivity equation proposed by Melcher (1979) is simplified case of ∂T/∂t = 0 (steady-state as T: temperature (K) at depth d (μm) and t: the time) assumed, the following equation may be obtained:
where Tm is the ambient temperature (K), T0 is the surface temperature of meteorite (K). In this case, the conduction of heat into a meteorite during flash heating may be estimated by approximating the meteorite surface as an infinite slab. Surface temperature of the meteorite might be at 1831 K, whereas the ambient temperature at 193 K (Rawer, 1984). Density of meteorite is 3.313×103 kg·m–3, thermal conductivity of 4.19 W·m–1·K–1 and specific heat of 8.24×102 J·kg–1·K–1 were employed for the calculation referring to thermodynamic properties of the orthopyroxene (Kobayashi, 1974). When the heating duration is assumed to be 5–20 seconds according to Sears (1975), we obtained temperature profiles across the meteorite (Fig. 6).
The result of CL examination suggests that zone 2 in the range of the depth from surface between ~200 and ~900 μm might be heated at around 1273 K, which is the transition temperature from Oen to Pen. Zone 1 was not heated up to 1273 K due to the presence of only Oen with a blue CL. Zone 3 between ~100 and ~200 μm might be expected to be heated up to the melting point of the enstatite at 1831 K due to the presence of HT-Cen supposed by the CL spectroscopy. The elevated temperatures in the zone estimated from the result of CL analysis might be consistent with the temperatures in corresponding depth from the surface calculated from the duration of 20 second (Fig. 6), which is able to be adapted due to allowable values compared to previously obtained ones (e.g., Sears, 1975). Therefore, this result supports the presumption of zonation mechanism due to phase transitions of the enstatite in Y-86004 during a flash heating when it entered the earth’s atmosphere. As mentioned above, CL imaging and spectroscopy are useful methods to provide valuable information on the crystal-chemical properties of the minerals occurring in terrestrial and extraterrestrial materials.