. Introduction
Reconstruction of the patterns of Pleistocene glaciations in the mountains of Southern Siberia, as well as correlating the sediments within mountains of Central Asia mountain belt and platform areas of Siberia, is not possible without absolute dating of glacial deposits, but these types of continental sediments are very complex to date absolutely. By now there is a wide arsenal of different numeric techniques for age determination of Quaternary sediments (Wagner, 1998). The application, precision and accuracy of each of them vary considerably (Fuchs and Owen, 2008).
However, within the Russian Altai utilizing most of techniques are highly problematic. Organic material generally is not presented in ancient glacial sediments. Moreover, glacial sequences are beyond the radiocarbon timescale and geological materials suitable for other radiogenic dating methods are also absent. Cenozoic formation of the Russian Altai was not accompanied by volcanic activity unlike to the mountain systems of the neighboring Tuva region where at least 5 glaciations were revealed for the last 1.75 million years (hyaloclastites, the products of subglacial volcuno eruptions were dated using the K-Ar method (Yarmolyuk and Kuzmin, 2006)). In contrast to Tuva, the effusive and tephra layers are absent in the sections of the Russian Altai. Repeated developing giant ice-dammed lakes in the region (Butvilovsky, 1993; Carling et al., 2002; Rudoy, 2005 and Herget, 2005) complicate utilizing terrestrial cosmogenic nuclide dating of ancient moraines within intermountain depressions. Besides the difficulties of applying the numerical dating techniques, in glacial sediments of Altai reference sections there is a lack of finds of macro– and microfauna (ostracods), and species composition does not allow establishing the glacial sequences. Palynological analysis is ineffective because of the significant redeposition of the pollen. Main reference sections in the Russian Altai also do not contain deposits that could be clearly and reasonably interpreted as interglacial ones. Thus, despite of more than 150-year history of investigations, the Quaternary of the Russian Altai is still poorly understood with the number and chronology of glacial cycles unknown and the proposed extent of glaciations varying widely between different authors.
Now thermoluminescence (TL) dates of glacial deposits in several areas of the Russian Altai form the basis for the formal Altai Pleistocene stratigraphic scale and depositional correlation schemes for the Russian Altai, Altai foothill plains, Western and Eastern Siberia (Volkova and Babushkin, 2000; Quaternary System, 2008 and Volkova et al., 2010). These data were obtained in the 1980s using the TL dating technique with artificially saturated etalons (Il’ichev et al., 1973 and Svitoch et al.,1978). Even then it was stressed that “taking into account the weak theoretical elaboration of the method, all given TL dates, should be regarded only for comparative analysis as relative measure of the deposits ages (older-younger)” (Svitoch et al., 1978, p. 16). The Chagan section, located in the southeastern part of the Russian Altai (SE Altai), is one of the most representative sections of the Altai Quaternary deposits. It includes more than a hundred-meter thickness of Pleistocene glacial sediments. A comprehensive study of this section was carried out in the 1970s and included TL dating (Svitoch et al., 1978). Eight TL ages, only three of which characterize the glacial and associated sediments in the upper part of the section, were taken for making geochronological reconstructions and first correlation schemes (Svitoch et al.,1978 and Arhipov et al., 1982).
Subsequently, a new set of absolute dates describing glaciations in this part of Central Asia was presented. For sediments of the Chagan section first TL dates utilizing naturally saturated etalons were published (Sheinkman, 1990) and several radiocarbon dates of carbonate concretions were obtained (Butvilovsky et al., 1996).
In this paper, we analyze tectonic and climatic factors that controlled the number and extension of the Pleistocene glaciations in different parts of the Russian Altai; present the results of our geomorphological investigations within the SE Altai with the main focus on the Chagan-Uzun river basin, where traces of different glaciations are preserved in topography; and finally compare these results with the results of applying different absolute dating techniques (previously published and obtained in this study) for dating glacial sediments of the reference Chagan section located within this basin. We also present the first experience of applying the IRSL method for feldspar from this section; discuss applicability of different luminescence techniques for dating the Pleistocene glacial sediments in the region and correlating these deposits with Siberian stratigraphic scales. The relevance of this work is evidenced by the interest of some international teams in studying and dating glacial features in Central Asia including Russian Altai applying various numerical techniques (Gribenski et al., 2014 and Stroeven et al.,2015).
. Regional setting
Regional oroclimatic features controlling the Pleistocene glaciations of the Altai intracontinental uplift
The Altai intracontinental uplift is a part of global watershed between the Arctic Ocean basin and the inland drainage basin of Central Asia (Fig. 1). The Altai Mountains are the northern part of the Central Asia collision belt. This mobile zone is clamped between Eurasia plate on the north and Djungar together with Tuva-Mongolian microplates on the west and on the east (Molnar and Tapponnie, 1975 and Novikov, 2004). As a result, the Altai intracontinental uplift stretches northwest more than 1500 km and forms a wedge shape narrowest in the southeast (about 50 km) and widest in the northwest (up to 500 km). The elevation increases in the opposite direction from 400 m a.s.l. to 4000 m a.s.l. In its northern part, the socle surface1 of the uplift is complicated by a sub-latitudinal steep scarp from 500 m high in the west to 1250 m in the east (Ufimtsev, 2002), which together with the other sub-latitudinal faults forms tectonic steps with altitudes about 1000, 2000 and 3000 m a.s.l. (Bogachkin, 1981). The modern climate of the region is determined by its intracontinental position, with the main humidity transfer from the west (Atlantic Ocean) and to a lesser degree from the north (Arctic), with a dominant influence of the Mongolian anticyclone, giving rise to increasing aridity southeastwards: annual precipitation decreases in this direction from 1000 mm in the northern part of the Lake Teletskoe depression (Selegey and Selegey, 1978), down to 150–200 mm within the bottom of the Chuya intermountain depression (Rusanov, 1961). This is resulted in complicated latitude-longitude orographic climatic zoning. This zoning is the most pronounced within the Russian Altai with its most complex structure for the whole Altai Cenozoic uplift. It determines glaciation patterns as well as varying preservation of ancient glacial landforms and deposits in different parts of the Russian Altai. Together with facies heterogeneity of deposits, interruptions in sedimentation, sparse distribution of reference sections, this results in different interpretations of amount, size and ages of the Pleistocene glaciations in the region. The problem of absolute age determination of glacial and associated sediments is another reason of inconsistency in existing views on this issue.
Within the Russian Altai the following areas, which are different in orographic and climatic features and therefore in manifestations of ancient glaciations, could be defined (Fig. 2). They are presented in a southward direction – in the direction of decreasing humidity and increasing altitudes (Fig. 3):
Fig 2
Neotectonic profile across Altai Mountains (See Fig.3 for profile location) and trends of average summer temperature and annual precipitation.
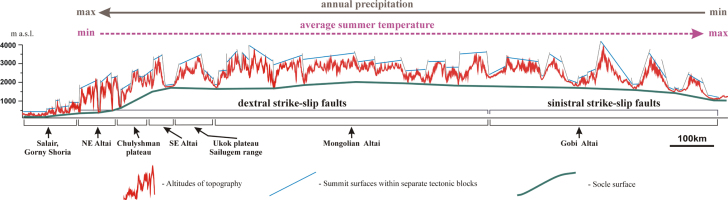
Fig 3
Location of the Russian Altai within the Altai Mountains. Star shows the location of the Chagan section.
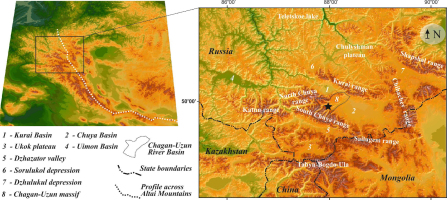
NE Altai, Lake Teletskoe area. There is Earth crust extension in this part of the Altai Cenozoic uplift (Novikov et al., 2004). Due to the graben of the Teletskoe Lake this area is characterized by significant difference in altitudes between summit (2000–2500 m a.s.l.) and socle surfaces: up to 1900–2200 m, with the lowest (for the whole Altai Mountains) altitude of the last one: about 250 m a.s.l. (average altitude of the lake bottom). Within mountain framing of the Teletskoe Lake tectonically driven topography roughness does not exceed 1000–1500 m. During the interglacial period in middle-late Pleistocene this area was affected by significantly lower tectonic uplifting (or even lowering) in comparison with the SE Altai. As a result, geomorphological evidence of ancient glaciations could be traced here at the lowest for the whole Altai altitudes: down to 900–1200 m a.s.l. (Agatova, 2005a). Besides the tectonic movements, moraines location at such low altitudes can be also explained by high precipitation in the region during the Pleistocene. Lehmkuhl et al. (2011) suggest that the Pleistocene ELA decreased here below 2000 m a.s.l. At about 1500–1600 m a.s.l. and higher the V-shaped valleys of lake tributaries are changed to U-shaped troughs with the morphologically younger kars at the heads of the valleys (Vysotsky, 2003). Nevertheless, some researches suppose that in the Middle Pleistocene (Svitoch et al., 1978 and Okishev, 1987, 2011) or in the end of the Late Pleistocene (Rudoy, 2013) the Lake depression was occupied by Teletsko-Chulyshman glacier with its feeding area located southward within the Chulyshman plateau. Baryshnikov (1992) supposes that the late Pleistocene glacier occupied only the northern part of the Teletskoe Lake depression and dammed ice melt water coming from the Chulyshman plateau. Further glaciers degradation triggered outburst flooding and lake drying at about 37.5 ka BP, which argue for maximal glacier advance in the area during MIS 4 – early MIS 3 (Baryshnikov et al., 2015). In river bank outcrops here Butvilovsky et al. (1996) distinguished evidence of four ancient glaciations — one occurred before the Pleistocene, one in the early Pleistocene and two others in the late Pleistocene (MIS 4 and MIS 2).
Chulyshman plateau, Sorulukol and Dzhulukul intermountain depressions (southern border of this area partly goes along the crest of the Kurai range). This area belongs to dextral strike-slip fault zone (Novikov et al., 2004) and is located on the steep slope of the socle surface with the altitude of about 500 m a.s.l. on the north up to 1800–2200 m a.s.l. on the south and 2200–2300 on the south-east. The tectonically driven topography roughness decreases in the same direction from 2000–2500 to 1000–1500 m, while altitudes of summit surfaces reach 2250–2300 in Sorulukol depression and 2800–3100 m a.s.l. in Chulyshman plateau. Development of mainly ice-sheet Late Pleistocene (Efimtcev, 1961; Devyatkin, 1965; Butvilovsky, 1993; Rudoy, 1995 and Lehmkuhl et al., 2011) and, probably, Middle Pleistocene (Okishev, 1982) glaciations in the region was controlled by relatively high precipitation level and low roughness of topography (Agatova, 2005a). The depth of the erosion incision during the interglacial period was about 100 m (Efimtcev, 1961). During the period of maximal development of the last ice-sheet glaciation ice flowed radially from several peaks, sometimes crossing the watersheds, while during the period of glacial degradation ice flow was controlled by valleys and local depressions (Butvilovsky et al., 1991 and Rusanov, 2009). Northern and northwestern direction of ice flow was determined by general inclination of the socle surface within this tectonic step. The last (MIS 2, Sartan according to the Siberia stratigraphic scale) from all Pleistocene glaciations is considered by most of researches as reasonably supported by absolute (radiocarbon and OSL) dates. At the same time, presence of erratic boulders and pebbles in brownish alluvium under moraines in some sections within northern slopes of the Kurai range suggests the existence of more old glaciation (Butvilovsky et al., 1991 and Butvilovsky, 1993). Most likely in this part of Russian Altai, which is characterized by relatively weakly dissected topography, glaciers during different Pleistocene glaciation epochs occupied practically the same area, and, therefore, mainly the surface effects of the last one could be detected in topography.
SE Altai, the Kurai-Chuya system of intermountain depressions and framing ridges except the Sailugem range (southern border of this area goes along the crests of the South-Chuya and Katun ranges). This is a transpressional zone formed due to oblique thrusting. It is located on the brow of the socle surface with an altitude of about 1500 on the west (Kurai depression) to 2000 m a.s.l. on the east (Chuya depression). The tectonically driven topography roughness is about 2600–3000 m with the maximal value about 3500 m within the Katun range. This is the largest value for the whole Altai Mountains. In spite of arid climate, due to the high altitudes of the ridges (up to 4506 m a.s.l.) the SE Altai is the centre of the modern glaciation. Geomorphologically distinct moraines of the maximal Middle- and two Late Pleistocene (Early Zyryanka (MIS 4) and Sartan (MIS 2)) glaciations are marked out here, last of which (Sartan) was the smallest one (Devyatkin, 1965; Okishev, 1982; Agatova, 2005b; Zol’nikov et al., 2010 and others). Troughs of the younger Pleistocene valley glaciation are cut into the troughs of the older valley and piedmont glaciation to about 500 m which is explained by uplifting and widening of the ridges by involving the peripheral parts of intermountain depressions into tectonic movements during the interglacial period (Agatova, 2005a, 2005b). Particularly in this part of the Russian Altai surface effects of different glaciations could be clearly distinguished in topography due to significant relief rebuilding during the interglacial period. Moraines on the watersheds, in intermountain depressions and in the valleys are confidently distinguished by their state of preservation. It could be adopted at least two main Pleistocene glaciation epochs for the SE Altai where there were sufficient altitudes for glaciers formation already in the Middle Pleistocene in contrast to other parts of the Russian Altai. Possibly the SE Altai is only area within the Altai Mountains system where this scenario was realized.
The presence of early Pleistocene glaciation within the SE Altai was suggested by (Svitoch et al., 1978) on the basis of first TL dates from the Chagan section, located at the northern foot of the South Chuya range, and by (Zykin et al., 2016) on the basis of finds of glacial boulders in brownish alluvial deposits under moraines within the southern slopes of the Kurai range. Analyzing OSL dates of glacio-lacustrine deposits in the Uimon intermountain depression, Zol’nikov et al. (2016) suppose MIS-5 stage (90–100 ka) for ice-dammed lakes formation there during Last Glacial Maximum. OSL ages of silt on the top of terminal moraines and in-between till deposits allowed Lehmkuhl et al. (2007) to date the last major ice advance in the SE Altai between 28 and 24 ka. Based on terrestrial cosmogenic nuclide dates of moraines in the Chagan-Uzun valley, Gribenski et al. (2016) suggested the Last Glacial Maximum in the SE Altai to be occurred about 17–19 ka. Associated with the glacial degradation final draining of ice-dammed lakes in the Chuya and Kurai intermountain depressions (Butvilovsky, 1993; Rudoy, 1995; Carling et al., 2002 and Herget, 2005) took place about 15.8 ± 1.8 ka, as it is evidenced by terrestrial cosmogenic nuclide dating (Reuther et al., 2006). The development of the Sartan ice-sheet glaciation within the framing ridges of the Chuya basin about 14 ka BP (Rudoy, 2005) does not confirmed by radiocarbon age of paleopeat in the Chikhachev range (Agatova et al., 2016).
South Altai, the South Altai and Sailugem ranges, the Ukok plateau (southern border of this area goes along the Tabyn-Bogdo-Ula range which is affected by modern glaciation). Socle surface of the main weakly dissected tectonic blocks and intermountain depressions lies at an altitude of about 1800–2300 m a.s.l., and summit surface — at about 3000 m a.s.l. Altitudes of the Tabyn-Bogdo-Ula range are 3200–3400 m a.s.l. with the highest peak at 4374 m a.s.l. That is an area of the Late Pleistocene low mobile valley glaciers and ice caps (Sailugem range) (Rudoy and Kirjanova, 1996; Galakhov and Samoylova, 2007) and ice reservoirs (high mountain depressions within Ukok plateau and Dzhazator tectonic valley, which took the glaciers from Tabyn-Bogdo-Ula and South Chuya ranges) (Okishev, 1982; Butvilovsky, 1993; Rudoy et al., 2000 and Derevyanko and Molodin, 2000). Geomorphological effects of older glaciations were not detected in the topography of this area.
This analysis indicates that SE Altai is the most perspective aria for studying the chronology of the Pleistocene glaciations.
Location of study site
The Chagan-Uzun river basin (Fig. 3) is located in the SE Altai and includes southwestern part of the Chuya intermountain depression and framing ridges. Tributaries of the Chagan-Uzun river are originated in the highest parts of the North and South Chuya ranges and in the flattened top of the Chagan-Uzun massif, which separates the Kurai and Chuya intermountain depressions.
Flower structure (Cunningham et al., 1996) is the dominant fundamental structure upon which the ranges of the SE Altai are constructed as a result of oblique thrusting (Novikov, 2004). Northern gentle and elongated slope of the South Chuya range consists of several tectonic steps and peripheral parts of the Chuya intermountain depression are involved into uplifting. One of the transverse tectonic riegels crosses the Chagan and Taldura valleys (up to 50 m in the Chagan valley). It controlled the Pleistocene glaciers development in these valleys (Ivanovsky, 1956 and Agatova, 2003).
Mapping accumulation surfaces of glacial origin within study area (Agatova, 2005b) revealed i) younger stadial moraine ramparts in valleys up to the nearest to modern glaciers LIA moraines; ii) two complexes (proximal and peripheral) of well-expressed terminal and lateral moraines in the Chuya depression; and iii) the oldest smoothed moraine cover partly preserved at watersheds on the lower tectonic steps of the range and in the intermountain depression outside the well-expressed peripheral moraine complex (Fig. 4). In the upper part of the Chagan-Uzun river basin younger glacial landforms are embedded into the older ones while they cover the older glacial landforms in the down part of the basin. Lateral moraines of both Taldura and Chagan peripheral moraine complexes merge on the Taldura-Chagan watershed at about 2600 m a.s.l. Studying denudation surfaces of glacial origin discovered a system of imbedded troughs including up to three generations, which could be correlated with accumulative glacial landforms.
Fig 4
Spatial distribution of glacial landforms and sediments of different states of preservation within the Chagan-Uzun massif. The cross-section across the Taldura-Chagan watershed (A-B) is shown below. Deposits of Miocene and Pliocene epochs are indexed as N11-3 and N21-2 correspondingly, and Pleistocene – as Q1-3 according to Stratigraphic Code of Russia (Zhamoida, 2006). Taking into account the lack of reliable absolute dates we follow the traditional Russian scheme of relative ages of ancient glaciations within the Russian Altai (Devyatkin, 1965; Okishev, 1982), where Q4 indicates the Holocene glacier advances, Q3 is associated with the late Pleistocene (Würm according Alpine scheme) including Q32 and Q34 – Zyryanka and Sartan stages according Siberian stratigraphic scheme correspondingly, and Q2 – middle Pleistocene (Riss according Alpine scheme). 1 – flattened and washed moraines, partly covered by sub-aerial cryogenic loams (Q2 ?); 2 – distal complex of well-preserved terminal and side moraines within Chuya depression, (Q32 ?); 3 – proximal complex of well-preserved terminal and side moraines, (Q34 ?); 4 – late Pleistocene – Holocene moraine ramparts of different glacial stages within river valleys, (Q34); 5 – LIA moraines, (Q4); 6 – glacial-lacustrine sediments of different ages; 7 – alluvial deposits; 8 – lakes and rivers; 9 – glaciers; 10 – altitudes (m a.s.l.); 11 – Chagan section; 12 – Chagan-Uzun River bounders. A-B – location of the cross-section across the Taldura-Chagan watershed.
Photos demonstrate moraines of different state of preservation and their locations (number in rectangle) within the basin.
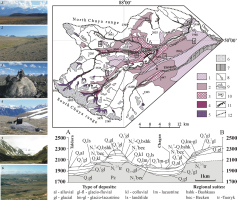
We suppose that washed and eroded remnants of the moraine covering outside the well-expressed terminal moraines on tectonic steps of the South Chuya range and in the Chuya depression are the traces of one (or possibly several) of the ancient piedmont glaciations. Complex of well-preserved moraines argue for valley type of the next glaciation. Stadial ramparts of the proximal moraine complex are younger because they are imbedded into moraines of the peripheral complex or cover them. Among all glacial landforms, moraines in the valleys are the youngest ones. Once again, the question of using them for reconstructing independent glaciation is open. Lack of geochronological data makes it difficult to determine the age of moraines except the nearest to the modern glacier LIA (13th–19th centuries) moraines (Agatova et al., 2012).
Therefore, different geomorphological features of various moraine complexes and their different state of preservation argue for at least of two ancient glaciations within the SE Altai. Most likely they were separated by prolonged interglacial period when due to tectonic activity the valleys of the South Chuya range were deepened.
Following the established traditional concept of the Russian Altai Quaternary geology (Devyatkin, 1965; Ivanovsky, 1967; Popov, 1972 and Okishev, 1982) we refer well preserved ramparts of proximal and peripheral moraine complexes to the Late Pleistocene glaciation, and washed and eroded remnants of moraine cover beyond these complexes — to the Middle Pleistocene glaciation. Thus, assuming these glacial sediments in the Chuya depression and at the watersheds on the lower tectonic steps of the South Chuya range belong to a single glaciation, in transitional zones where younger sediments cover the older ones, we can expect moraine complexes at least of two glaciations to be exposed in proximal outcrops including the presented here Chagan section.
Chagan Section: description and available data
The Chagan section, located in the transition zone between the Chuya intermountain depression and South Chuya range (Fig. 4), is one of the reference sections of the Pleistocene glacial, glacio-fluvial, and glacio-lacustrine deposits in the region. This section is about 4 km long and ~200 m high containing several units (Fig. 5). Interaction of the Chagan and Taldura glaciers in the area of their conjunction determines the structural complexity of glacial thickness here. In the Chagan river valley lateral ramparts of the proximal moraine complex within the upstream part of the section are at about 2250 m a.s.l. Downstream the altitude of the section increases due to overlaying lateral moraines of the Taldura glacier.
Fig 5
Chagan section – the regional reference sections of the Pleistocene glacial, glacio-fluvial, and glacio-lacustrine deposits. Aerial photograph A) and common view of the section B). Arrow shows the location of the studied section (Fig. 7). Regional suites and types of deposits are described in Fig.4.
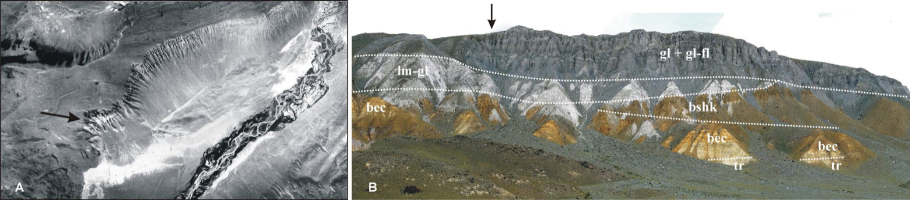
Preglacial deposits at the bottom of the section have Low Neogene — Low Pleistocene ages (Devyatkin, 1965 and Rusanov, 2011). They include light gray and yellowish-gray lacustrine sediments of the Tueryk suite, yellowish-brown lacustrine-alluvial sediments of the Beken suite, and brown-reddish alluvial sediments of the Bashkaus suite. We have also explored sediments of the Tueryk suite (middle Miocene — middle Pliocene) in structure of the Chagan-Taldura watershed when studying the giant landslide triggered by the 2003 Chuya earthquake (MS = 7.3) opposite the Chagan section in Taldura river valley (Fig. 4) (Agatova et al., 2004).
Overlying glacial deposits have a basal thin (1.5–7.0 m) moraine layer with brownish redeposited sediments of the Bashkaus suite.
Above it there is a lens (up to 45–50 m) of light gray fine-grained glacio-lacustrine sediments with drop-stones and layers with carbonate concretions (up to 10–15 cm in diameter) at the unit boundaries. Svitoch et al. (1978) and Butvilovsky (1993) suggested the duration of this lens accumulation about 130–150 years. Some researchers believe that it was a periglacial lake at the glacier margin (Glushankova and Voskresenskaja, 2008) or moraine-dammed lake (Sheinkman, 1990), whilst others think that these sediments fill the paleo river channel produced in Bashkaus pebbles (Rusanov, 2011). This paleochannel stretches along the foot of the South Chuya range i.e. across the modern Chagan-Taldura watershed and across the Chagan section. Rusanov (2011) supposes that the lacustrine sediments of this lens were accumulated in the narrow bay of the Pleistocene ice-dammed lake in the Chuya depression. When studying the surface effects of the 2003 Chuya earthquake we did not find any evidence of the possible continuation of this channel (lake bay) at the opposite side of the Chagan-Taldura watershed in the main scarp of giant seismically induced landslide in the Taldura river valley.
Above the lens there is a thick (about 60–80 m) gravel-dominated section of gray moraine and glacio-fluvial sediments with distinct sandy layers of fluvial origin. The glacial deposits are characterized by a high degree of facies variability, which cause difficulties in distinguishing the different glacial episodes. Before the 2003 Chuya earthquake two clearly distinguished steps were formed as a result of long-term erosion. Devyatkin (1965) interpreted them as evidence of two different glaciations but it could be also explained by different resistance of strata to erosion. Borisov (1984) and Butvilovsky et al. (1996) establish five separate glacial events in this section but these reconstructions are not correlated with each other.
The top of the exposure has a height of 2250 m a.s.l., which coincides with the possible maximum filling of the Pleistocene ice-dammed palaeolakes in the Chuya depression (Rusanov, 2008). The timing of formation and the outburst events of these giant lakes is still debated, however, the preservation of high lake terraces here argues for moraines formation before the maximum occupancy of the lake. Thus, the age of the largest ice-dammed palaeolake could be assumed as an upper possible age of deposition processes. It could be correlated with the late Pleistocene (25–12 ka BP (Rudoy, 2005 and Rusanov, 2008)) or, in contrast, with the middle Pleistocene (Zol’nikov and Mistrukov, 2008). We assume the formation of the Chagan section as a result of lake abrasion and seismically induced landsliding. The entire Chagan section was exposed by repeated earthquake-triggered landslides. Seismic origin of this multi-kilometers outcrop in permafrost rocks was confirmed during the 2003 Chuya earthquake. A number of new seismo-gravitational cracks were developed along the brow of the valley slope. High seismicity during the accumulation of sediments in this section is evidenced by numerous seismically induced disturbances: seismic convolutions, cracks and offsets (Agatova et al., 2006). Some landslides, composed of Bashkaus alluvial pebbles and covered by thin washed moraine, were erroneously taken as moraines of the Akkem glacial stage (one of the Late Pleistocene stages of glaciers advances in the SE Altai) by Borisov (1984), but this interpretation is adopted in the Russian Altai stratigraphic scheme.
Paleomagnetic characteristics of the Chagan section argue for two short-term episodes of magnetic polarity reversals for glacial part of this section (Faustov et al.,1971 and Svitoch et al., 1978). One of them, Chagan episode, corresponds to the lens of glacio-lacustrine deposits and has TL age 266 ± 30 ka. The second one, correlated with Blake geomagnetic polarity episode, has TL age 145 ± 13 ka. It was established for glacial deposits at the depth of about 20–45 m and probably could serve as a reference layer for age distinguishing of moraines. At the same time, as it was mentioned by (Svitoch et al., 1978), correlation of obtained paleomagnetic zones in the Chagan section with the paleomagnetic chronological scale is very difficult. Now the age of the Chagan episode (Biva II) is assumed to be about 290 ka (Quaternary System, 2008).
First palynology studies of the Chagan section (Svitoch et al., 1978) revealed similarity of spore-pollen spectra from this section and those obtained in areas affected by modern glaciation. However, we have twice failed to detect pollen in glacial deposits of the Chagan section (Mikhailova et al., 2004). Generally due to intensive pollen transportation and redeposition, palinological analysis of glacial deposits is not informative and should cautiously be used only in complex with other techniques.
There are also only single paleontological finds in this section (Mikhailova et al., 2004 and Rusanov and Vazhov, 2014). Generally, all applied geological and geophysical methods did not allow making chronological distinguishing of glacial sedimentation in the Chagan section.
The first absolute dates for the Chagan section were obtained in the late 1970s. The Early and Middle Pleistocene ages of moraine deposits from Chagan section were based on three TL dates – 145 ± 13, 266 ± 30 and 476 ± 51 ka obtained with artificially saturated samples (Fig. 6) (Svitoch et al., 1978). They suggested the Middle Pleistocene glaciation as being the largest one whilst, in spite of more significant lowering of the snow line, the Late Pleistocene glaciation (which is not presented in the Chagan section) is thought to be just a valley glaciation. Until now these dates have formed the basis of the formal region correlation schemes for the Russian Altai (Volkova and Babushkin, 2000; Quaternary System, 2008 and Volkova et al., 2010). At the same time, all recent editions of these schemes applied a concept of Borisov (1984) as a basis. Using the same TL dates, he described much more glaciations in deposits of the Chagan section (one Early, two Middle and two Late Pleistocene glaciations, as well as tree interglacial periods).
Fig 6
Correlation scheme of absolute dates for glacial sediments from Chagan section obtained by different authors. Designation of deposition units is given in Fig. 7.
* No information about both the dating laboratory and sample codes was presented by the author. First mention about these dates was given in 90th of the last century (Sheinkman, 1990, P. 81). Twelve years later these dates were recalculated (Sheinkman, 2002, P. 45, 46) (without any explanations of methodology or the reasons) and their position in the section was specified (underlined dates). This could not allow utilising these data as the “new generation dates”, as they were presented in (Zol’nikov and Mistrukov, 2008 and Rusanov, 2011)
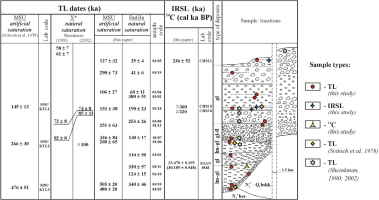
At the end of the 20th century there was an attempt to revise the initial TL data using a TL technique based on applying naturally saturated etalons (Sheinkman, 1990; 2002)2; however, the attempt to date the lowest glacial strata failed. Five new TL ages for the Chagan section were obtained; one sample from the upper part of the lens of glacio-lacustrine sediments which gave age ≥ 100 ka, and four from glacial deposits in the upper part of the section which fell into the range of values 58 ± 7 – 85 ± 11 ka (Fig. 6). Three more TL dates (81 ± 10, 121 ± 14, and 135 ± 15 ka) were obtained from another separate outcrop located 3 km upstream the Chagan river (see Discussion for comments). Sheinkman (2002) proposed a Late Pleistocene age for these glacial sediments and believed the Late Pleistocene glaciation being the largest one within the Russian Altai.
There are also three radiocarbon dates of carbonate concretions from the glacio-lacustrine lens at the bottom of the glacial part of the section and from thin glacio-lacustrine layers in its middle part (Fig. 6). They were obtained by Butvilovsky (Rusanov and Orlova, 2013). Radiocarbon samples have an inverse stratigraphic position in the section (the older samples are located above the younger ones) and their ages have another order of magnitude in comparison with the TL data. These dates fall into the Sartan glaciation (the end of the Late Pleistocene).
Rusanov (2011) marks out two units in the glacial part of the Chagan section. The low unit includes the basal moraine and glacio-lacustrine lens when the upper one is the single gray-colored unit of about 100 m thick. With the azimuthal unconformity the upper unit overlays the eroded surface of the lens and alluvial gravels of the Bashkaus suite. At the bottom of this upper gray-colored unit a single paleontological find (heavily damaged section of the upper tooth - Equus sp., presumably of the late Pleistocene age) was discovered by Rusanov (2011) and he suggests the Late Pleistocene age for the whole upper 100 m unit.
The significant discrepancy between TL dates based on different techniques as well as their disagreement with radiocarbon dates prompted us to carry out our own investigation of glacial and associated sediments of the Chagan section including applying different absolute dating techniques (radiocarbon, TL and OSL methods).
. Absolute dating of glacial and associated sediments from the chagan section
Radiocarbon dating
A single sample for radiocarbon analysis was collected from carbonate concretions in glacio-lacustrine lens located at the bottom of the glacial part of the section above the brownish sediments of Bashkaus suite (Fig. 7).
Sample preparation and radiocarbon age measurement was made at the Institute of Geology and Mineralogy SB RAS, Novosibirsk. The determination of carbon residual activity was done with the QUANTULUS-1220. The conventional radiocarbon age was calibrated (2-sigma standard deviation) applying the CALIB Rev 5.0 program (Stuiver and Reimer, 1993), with the IntCal09 calibration data set (Reimer et al., 2009).
The calculated age (30189 ± 548 cal. BP; SOAN 8548 (Table 1)) correlates with the other available radiocarbon data previously obtained at the same laboratory by Butvilovsky (Rusanov and Orlova, 2013).
Table 1
Obtained absolute dates.
Applied techniques: RTL – radiotermoluminescence; IRSL – Infra Red stimulated luminescence; 14C – scintillation radiocarbon analysis (both calibrated (2σ) and radiocarbon (in brackets) ages are given).
Luminescence dating
Sampling strategy
Insufficient bleaching of glacial sediments is a common problem for making adequate luminescence dating (Fuchs and Owen, 2008). Deposits associated with proglacial, glacio-fluvial and glacio-lacustrine sediments have a higher potential to be bleached than subglacial and englacial ones, but the risk of insufficient bleaching is still considerable. For example, glacio-fluvial sediments may not be fully exposed to daylight due to the turbidity in the meltwater or attenuation of the daylight intensity with depth. Modern glacio-fluvial samples have been shown to exhibit residual luminescence signals which lead to overestimation of the calculated ages (Gemmell, 1997; 1999). Essentially, the effectiveness of luminescence signal zeroing is directly controlled by the geomorphic processes responsible for the sediment production and reworking. Thus, a geomorphological under standing of the glacial environment is required to identifysediments and the association with the particular geomorphological process in the context of sufficient intensity and duration of daylight exposure.
Prior to sample collecting, detailed geomorphological investigations of the Chaga-Uzun river basin were carried out in order to determine the number, patterns of and evolution of the Pleistocene glaciations in the region.
The identification of appropriate sediments for luminescence dating, as well as samples collection, was done following recommendations and suggestions of Richards (2000) and later of Benn and Owen (2002). The material for luminescence dating was derived from glacio-fluvial, glacio-lacustrine and englacial meltwater sediments to estimate the age of moraine formation equal to the age of glaciations. Several samples were collected from alluvial deposits of the Bashkaus suite, which underlies the Pleistocene complex of glacial deposits in the Chagan section, to estimate the lowest possible age of glaciations. Preference was given to deposits containing finely grained fractions. During the later field researches in situ doserate measurements were carried out by portable gamma ray spectrometer.
Thermoluminescence dating
TL method was used prior to the development of OSL and IRSL methods. Previously, the applicability of TL dating has been demonstrated for various sedimentary environments (Wagner, 1998 and references therein), mainly focused on those that have experienced sufficient exposure to daylight enabling a complete resetting of the luminescence signal. Luminescence dating of glacial and associated sediments is difficult, mainly due to insufficient bleaching during transportation and deposition of the sediments (Fuchs and Owen, 2008 and references therein).
In addition to the problem of zeroing of the luminescence signal, there is also a great regional diversity in physical properties of quartz grains. Quartz properties, with regards to their luminescence signal, are mainly determined by the content and composition of microimpurities which form electron traps in the mineral crystal structure. This leads to variations in the suitability of a quartz or alternative silicate minerals (such as feldspar) as a geochronometer for luminescence dating.
Generally 38 samples were collected from deposits containing sands and sandy loams within the Chagan section. Luminescence sample preparation was carried out under subdued red light applying standard chemical treatment including clay fraction washing and wet sieving (0.10–0.25 mm), hydrochloric and hydrofluoric acid etching, ultrasonic grinding with further sieving (0.1 mm), and separation of the light fraction in bromoform. Thermoluminescence dating was carried out in two laboratories which applied different radiothermoluminescence (RTL) methods. The RTL dating technique with the artificially saturated standard (Vlasov and Kulikov, 1989) was applied in the Laboratory of dosimetry, radioactivity and RTL dating, Moscow State University (MSU), while RTL age determination using naturally saturated standard (Shlukov et al., 1997 and Perevalov and Rezanov, 1997) was utilized in the RTL dating Laboratory, Buryatia Geological Institute SB RAS (BuGIN). Considering the problems associated with the applying of luminescence methods for dating glacial and associated sediments, as well as the complexity of the samples used for testing these techniques, we sent five samples to both laboratories.
20 new RTL dates are presented in table (Table 1, Fig. 7). Among all studied samples there were three naturally saturated ones, which is crucial for age measurement when applying the RTL technique on the basis of naturally saturated etalons (Shlukov et al., 1997).
11 BuGIN RTL dates (obtained with the naturally saturated etalon) indicate accumulation of glacial deposits in the Chagan section between 25 ± 4 – 380 ± 51 ka while nine MSU RTL dates (obtained with the artificially saturated standard) define this time interval as 106 ± 27 – 505 ± 20 ka. At the same time four among five samples dated in both laboratories return different ages. Moreover, there is a discrepancy with the stratigraphic position for samples dated in both laboratories. The highest correlation amongst all dates is observed for the lens of glacio-lacustrine sediments. Five dates are in the range of 260–340 ka (the Middle Pleistocene) and only one (obtained in MSU as 505 ± 20 ka, which was also dated in BuGIN as 330 ± 57 ka) stands out.
It is noteworthy that in the samples analyzed at BuGIN, from the 24 successfully prepared samples a TL signal was measured only from 14 samples, including the 3 saturated ones. Quartz extracted from the remaining ten samples gave powerful low temperature peak and therefore was unsuitable for measurements. A similar proportion of undated samples came from MSU laboratory. This fact, once, emphasizes the importance of the discussion about choosing the mineral-paleodosimeter (quartz versus feldspar) for regional luminescence dating.
OSL dating
The optically stimulated luminescence (OSL) method is expected to be more suitable for dating glacial sediments, mainly due to the significantly shorter time (several seconds) of daylight exposure needed for complete bleaching the sample. In 2008 we made a first attempt to apply this technique for dating deposits of glacial origin from the Chagan section. A number of samples were taken from the glacio-fluvial and glacio-lacustrine layers. It was discovered that quartz in these samples showed a strong response to IR stimulation, thought to be resulting from feldspar present as inclusions, and therefore feldspar had to be used for analysis although it is only present in very low quantities.
Initial tests on the IRSL signal obtained at 50°C stimulation temperature demonstrated relatively high De values (>300 Gy) and fading rates of up to 7%. This combination of a large natural De and high fading rates led to problems when applying a fading correction, as the corrected De value falls on the “flatter” part of the dose-response curve near/in saturation. For age estimation of the samples, the post-IR IRSL dating procedure (Thomsen et al., 2008 and Buylaert et al., 2009) was applied using IR stimulation temperatures of 50°C and 225°C. Using this protocol, a number of the older samples had a post-IR IRSL De value in saturation, and typical fading rates of between 0 and 2% were measured (Agatova et al., 2014). Applying the fading correction to the De values obtained from both the 50°C IRSL and post-IR IRSL signal resulted in similar De values where the results were not in saturation. Additionally, the residual doses in both the 50°C IRSL and post-IR IRSL were measured following sunlight bleaching of untreated discs from sample CHM1 (uncorrected De of ~300 Gy) and appeared to reach a plateau of ~5 Gy and ~20 Gy, respectively, after 1 hour.
Applying this technique 3 absolute dates were obtained in Institute for Geology and Palaeontology, University of Innsbruck, Austria (Fig. 7). The Middle Pleistocene age (236 ± 52 ka (CHM1)) of the glacio-fluvial sandy layer in the upper part of the section at 2250 m a.s.l. was obtained. It is older age than all previously published dates for the upper part of the section. Geochemical analysis reveals a significant difference in content of petrogenic oxides between this layer and other glacial stratums, suggesting a possible change the source area and pattern of sedimentation in the upper part of the section. A glacio-fluvial sandy layer at a depth of about 60 m has a Middle Pleistocene age or older (>360 ka (CHM3), >220 ka (CHM8)).
. Discussion
The numerical age estimation for deposits of the Chagan section is important for the regional stratigraphy and region correlation schemes for the Russian Altai and Siberia. At the moment, there are 4 radiocarbon ages (three previously published), 28 TL (8 previously published) ages, and 3 IRSL ages for the upper part of the section, which includes glacial sediments of various types; and 2 previously published TL dates for underlying alluvial deposits3.
Taking into account the physical basis of the applied luminescence techniques, OSL method is expected to be more reliable in comparison with TL. It has the advantage shorter times needed for complete bleaching of the luminescence signal, which is particularly important when dating glacial and associated sediments; it takes just a few seconds of direct sunlight exposure for the more light sensitive OSL and IRSL signals against several tens of hours for less light sensitive TL signal.
Analysis of all the available luminescence dates revealed the best correlation of results in the lens of glacio-lacustrine deposits and allows to assume its Middle Pleistocene age, that, however, requires further verification.
We also argue that it is inappropriate to correlate aleuropelite layer from glacio-lacustrine deposits in the Tedesh section (TL dates 121 ± 14 and 135 ± 15 ka were reported for these sediments in (Sheinkman, 2002)) with glacio-lacustrine lens in the Chagan section, and therefore, to utilize these TL dates for absolute age estimating glacio-lacustrine deposits in the Chagan section as it was done in (Sheinkman, 2002; Zol’nikov and Mistrjukov, 2008 and Rusanov, 2011). Such geochronological correlations are not valid, regardless of the applied dating technique. Tedesh and Chagan sections are located in 3 km from each other and are separated by the transverse tectonic riegel crossed the Chagan valley. Slope in this part of the valley is covered by talus fans with no clear transition between these sections and glacio-lacustrine sediments of the lens wedge out at the surface of the tectonic riegel.
Good correlation of TL dates for the glacio-lacustrine lens supports the thesis that glacio-lacustrine sediments are one of the most perspective ones for luminescence dating among all glacial and associated deposits. It is likely that the sedimentation patterns in the glacial lake increased the extent of bleaching when compared to the sedimentation during subglacial and englacial processes. The possible Middle Pleistocene age of this lens also does not contradict to the results of our geomorphological investigations, which argue for presence of deposits of several glacial epochs in the Chagan section. We suppose that following researches should pay special attention to this lens in the Chagan section while making further study and testing various numerical techniques for dating glacial and associated sediments.
Generally, the large scattering of obtained TL ages and their low correlation with the stratigraphic position in the section allow us to conclude that TL method does not fit for dating glacial sediments and landforms, and cannot be used for stratigraphic breakdown, as well as for making any geochronological reconstructions and correlations.
The OSL technique is expected to be more suitable for dating glacial sediments. Narama et al. (2007) reported the successful application of the OSL technique for dating glacial and loess deposits in the northern flank of the Terskey-Alatoo Range, Central Asia during the Last Glacial. Material for the OSL dating (fine- and coarse-grained quartz) was collected from loess and redeposited glacial sediments with the fluvial layers at up to 2 m depth. Obtained OSL data were partly controlled by radiocarbon method and indicated glacier expansion between 29 and 21 ka BP during MIS2 with the maximum glacier advances occurred at about 76 ka BP during the MIS4. Good OSL dating results were also obtained for supraglacial meltout tills in Nepal (Tsukamoto et al., 2002), and for glacio-fluvial deposits, which included sand lenses sandwiched between tills in moraines (Zhao et al., 2013).
At the same time glacial sediments within the Russian Altai are very problematic for luminescence dating. Applying OSL dating technique for fluvial and aeolian deposits Lehmkuhl et al. (2007) show the last major glaciers advance in the Russian Altai between 28 and 24 ka in the beginning of the last glacial cooling maximum followed by an increased rate of loess accumulation. IRSL dating approach (utilized on moraines and laminated ice-dammed lake sediments) yielded low signal intensities and maximum ages with large uncertainties owing to insufficient bleaching during short distance transport (Lehmkuhl et al., 2007). OSL dates of glacio-lacustrine deposits within the Chagan-Uzun river basin 5 km downstream the Chagan section scatter between 200 and 50 ka and are not in stratigraphic order. Same difficulties in applying luminescence techniques for dating glaciofluvial deposits in Estonia were reported by (Raukas and Stankowski, 2005).
The small number of IRSL dates obtained does not allow us to assess the appropriateness of using this method for age estimation of glacial and associated sediments, which remain one of the most difficult types of continental deposits for dating. It also does not allow clearly determine the ages of glacial deposits in the Chagan section. We can only assume a relatively low number of depositional cycles for the grains in this section and a short transport which is also problematic for luminescence dating techniques.
Another important problem is the selection of the mineral-dosimeter and associated task of choosing the best dating technique. Among two the most commonly used minerals, quartz and feldspar, the luminescence signal from feldspar is an order of magnitude greater than for quartz (Aitken, 1998). Feldspar, however, is less rapidly bleached than quartz, sometimes by a factor of about 10 (Godfrey-Smith et al., 1988), and also suffers from the problem of anomalous fading (Aitken, 1985, 1998).
Our study revealed that strong low temperature peak in the TL signal is a regional feature of quartz from the Chagan section. This led to failure in determining a number of TL ages. It was also discovered that regional quartz showed a strong response to IR stimulation, thought to be resulting from feldspar inclusions. In general, the mineral material from the Chagan section is not ideal for luminescence dating. This is probably due to a combination of a short transportation distance and a low number of depositional cycles the grains have undergone since they were eroded from bedrock.
Further studies of regional minerals-dosimeters should be undertaken in order to sort out the most effective luminescence dating techniques of glacial and associated sediments in the SE Altai. The small number of IRSL dates obtained does not allow us to fully evaluate appropriateness of this technique for absolute age estimation of glacial sediments in the Chagan section.
Radiocarbon dates of carbonate concretions from the bottom and the middle part of the glacial complex in the Chagan section generally correlate to each other: 22946 ± 482 cal. BP (SOAN 3115), 22264 ± 657 cal. BP (SOAN 3116), 24857 ± 470 cal. BP (SOAN 3117) (Rusanov and Orlova, 2013), and 30189 ± 548 cal. BP (SOAN 8548) (this study). It should be mentioned that this type of material is not easy and comfortable for absolute dating. Some difficulties of applying radiocarbon method for carbonate concretions dating have been previously discussed (Aitken, 1990 and Bowman, 1995). Problems of radiocarbon age measurements for lacustrine carbonate samples from the Pleistocene Lake Lahontan, Nevada, USA are presented by Lin and coauthors (Lin et al., 1996). They reported systematically younger ages and suggest it arises as result of problems of contamination with secondary calcite and the local reservoir age correction.
Radiocarbon dates of carbonate concretions argue for the relatively young age of their formation. An inverse stratigraphic sample position in the section (the older samples are located above the younger ones) could be explained by intensive Chagan river incision into the previously accumulated glacial deposits at the end of the Late Pleistocene during the interglacial time (Butvilovsky, 1993).
Heavily damaged section of the upper tooth (Equus sp.), presumably of the late Pleistocene age, is a single palaeontological find which was done at the bottom of upper part of the section about 100 m below the surface (Rusanov, 2011). This fragment, especially taking into account the state of preservation, is insufficient to determine the age of inclosing sediments (Foronova I.V., IPGGP SB RAS, personal communication).
Thus, it could be stated that number of glaciations associated with the glacial sediments of the Chagan section (Borisov, 1984 and Quaternary System, 2008) is overestimated and their chronology is not currently proved. A similar conclusion was also made by Zol’nikov and Mistryukov (2008) and Rusanov (2011). Deposits, previously interpreted as Akkem moraines (the end of the Late Pleistocene) (Borisov, 1984), are actually landslide which is composed of alluvium sediments of the Bashkaus suit. Only on top it is clothed by a thin moraine cover. Generally by now the Chagan section could hardly serve as a reference section for the Altai stratigraphy. Further geochronological researches based on modern absolute dating techniques are needed to make stratigraphic breakdown and correlations.
. Conclusion
Our detailed geomorphological investigations argue for presence at least two major Pleistocene glaciations in a region. These results support a scheme of glaciation history within the SE Altai suggested by Devyatkin (1965) and Okishev (1982). Analysis of all available numerical age estimations of glacial and associated deposits for the reference Chagan section, SE Altai, indicates that glacial environment is one of the most complex for absolute dating.
The presented luminescence ages, obtained with both naturally and artificially saturating TL techniques showed the best data correlation in the lens of glacio-lacustrine sediments at the bottom of glacial part of the Chagan section and suggested its possible Middle Pleistocene age. The age of basal moraine as well as the time of glacial sedimentation above the lens is not reliably established. Numerous TL dates revealed a large spread in the obtained ages measured in different laboratories on the basis of various techniques. The dates sometimes poorly agree with the stratigraphic position of the sample in the section. The obtained results demonstrate that TL method is not suitable for dating glacial sediments and available TL ages cannot be treated as geochronological markers. It should only cautiously be used for making any reconstructions and correlations.
Three IRSL dates indicate Middle Pleistocene age in the upper part of the section. The small number of obtained IRSL dates cannot form the basis for geochronological reconstructions of the Pleistocene glaciations in the SE Altai, but gives the possibility for further experiments with different variation of OSL (IRSL) techniques.
The available radiocarbon dates of carbonate concretions from this section are significantly younger and indicate the age inversion of their formation. Generally, they fall into interglacial period before Sartan glaciation and indicate the probable Chagan river incision into the ancient glacial deposits which were accumulated long before.
Analysis of the physical properties of minerals-dosimeters reveals specific regional quartz features such as strong low temperature peak in TL signal which often makes it impossible to measure luminescence at chronometrical peak near 300°C. Strong response to IR stimulation is provided by feldspar inclusions. Relatively low number of depositional cycles for the mineral grains in this section and a short transport distance was established. Thus, further experiments on selecting the appropriate mineral and luminescence dating technique are still relevant.
It could be stated that by now the age of Pleistocene glacial landforms and sediments within the Russian Altai remains a mainly relative determination and such age markers as “Late” or “Middle Pleistocene” mean mainly relative terms “older” — “younger”. The large spread in ages of deposits in reference sections within the Russian Altai and the weak provision of some of them with absolute dates do not allow the correlation of glaciations between different oroclimatical zones of Russian Altai, or further comparison with the other mountain systems of Central Asia and Siberia. The adopted scheme of correlation between Altai and Siberia glaciations, based on the first TL dates of the Chagan section, needs to be improved.