. Introduction
Dendrogeomorphology has lately become a recognised and useful tool in dating past mass movement activity (e.g. Shroder, 1978; Lang et al., 1999; Stoffel, 2010; Franco-Ramos et al., 2017; Malik et al., 2017), including landsliding. Tree-ring analyses of landslide activity cover such topics as the dating methods (e.g. Braam et al., 1987; Malik and Wistuba, 2012; Wistuba et al., 2013; Šilhán and Stoffel, 2015; Šilhán, 2017), magnitude-frequency relations (e.g. Stefanini, 2004; Corominas and Moya, 2008, 2010; Malik et al., 2016), landscape evolution (e.g. Migoń et al., 2014; Wistuba, 2014; Wistuba et al., 2015), triggering factors (e.g. Paolini et al., 2005; Šilhán et al., 2012; Łuszczyńska and Wistuba, 2015; Papciak et al., 2015; Cockburn et al., 2016), as well as topics related to the potential of landslide reactivation (e.g. Lopez Saez et al., 2012) and landslide hazard assessment (e.g. Łuszczyńska et al., 2018).
Since dendrochronological analyses of landsliding can be also used for practical purposes, particular attention should be paid to the accuracy of dating and its improvement. Nevertheless, ground movement is only one of the many environmental factors recorded by trees. The width and anatomy of every seasonal ring of wood reflect the ontogenetic properties of a certain tree as well as the complex conditions of tree growth in a certain location, in a certain growing season (Fritts and Swetman, 1986). The dendrochronological record obtained from trees growing on landslides can be, therefore, also affected by other geomorphic and non-geomorphic phenomena such as soil creep (e.g. Šilhán, 2017), avalanches (e.g. Tumajer and Treml, 2015), air pollution (e.g. Malik et al., 2012), insect outbreaks (e.g. Wang et al., 2017), wind (e.g. Duncker and Spiecker, 2008) and other climatic factors (temperature, precipitation; e.g. Nöjd et al., 2017), etc. Disentangling the dendrochronological record of landslide activity from the record of the above-listed factors is all the more difficult because it is not landsliding itself that trees record but the tilting of stems (Braam et al., 1987) (Fig. 1A). According to Wiedenhoeft (2013), the tilt of a tree stem from the upright orientation by as little as 1–2° causes enough stress to disturb the normal radial growth of wood. In coniferous trees radial growth and cell differentiation are accelerated on the lower sides of tilted stems (Shroder Jr, 1978; Braam et al., 1987) where the development of eccentric growth (characterised by the increased width of rings on one side of a stem compared to the opposite side: Braam et al., 1987; Wistuba et al., 2013) and the formation of compression wood (characterised by thicker and more rounded cell walls, smaller cell lumens and the presence of intracellular spaces – Ruelle, 2014) begin immediately after tilting (Timell, 1986).
Fig. 1
Habits and eccentricity of annual rings in Norway spruce (Picea abies Karst.) trees: spruce tilted upslope due to landsliding (A) and spruce tilted leeward due to prevailing wind (B). Direction of stem sampling marked: u– upslope radius, d– downslope radius, w – windward radius, l– leeward radius.
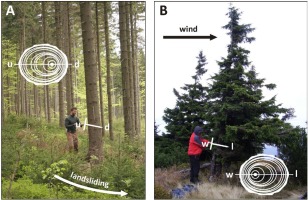
Owing to these advantages (immediate reaction and high sensitivity), eccentricity and compression wood are often used for landslide dating (e.g. Braam et al., 1987; Lopez Saez et al., 2012; Šilhán et al., 2012; Wistuba et al., 2013; Migoń et al., 2014; Cockburn et al., 2016; Malik et al., 2016; Šilhán, 2017; Łuszczyńska et al., 2018). However, other environmental factors, e.g. soil creep, avalanches and wind, can also tilt tree stems and result in the production of eccentricity and reaction wood. Proper dating requires the elimination of such non-landslide impact from the chronologies developed for the purpose of reconstructing landslide activity. This elimination can be obtained e.g. by using reference thresholds in the dating procedure (Wistuba et al., 2013). This approach to dendrochronological dating assumes that only dendrochronological events which exceed the average level of growth disturbance found on a reference slope can be recognised as landsliding events. Reference slopes are selected as slopes devoid of landslide activity which have, however, other environmental features similar to the landslide slopes under study (Wistuba et al., 2013).
Dating precision can also be improved through appropriate determination of the growth patterns developed as a result of diverse environmental factors, among which wind is one of the strongest. The prevailing wind (wind from one prevailing direction) can affect trees particularly severely. The constant impact of the prevailing wind can result in flagging (a change in the growth habit of tree crowns) (Fig. 1B) and even developing bush or shrub growth habit by species which usually have a tree-like growth habit (Schweingruber, 1996). Deformed growth habits result from the gradual adaptation to continuous unfavourable wind conditions (Ennos, 1997) which is also recorded in tree rings and wood anatomy (Schweingruber, 1996). Duncker and Spiecker (2008) have analysed tree-ring eccentricity and compression wood developed by coniferous trees affected by the prevailing wind. However, so far there have been no comparisons between growth disturbances caused by the prevailing wind, disturbances caused by landsliding, and normal radial growth. Therefore, the aim of our study was to compare patterns of tree-ring eccentricity developed as a result of landsliding (the Suchawa study site in the Kamienne Mts.), prevailing wind (the Hubertka study site in the Hrubý Jeseník Mts.) with one another and with the normal radial growth of trees unaffected by landsliding or the prevailing wind (the Suchawa and Hubertka reference sites in the Kamienne Mts. and in the Hrubý Jeseník Mts.).
. Study area
The Suchawa study site and reference site
The Suchawa study site and reference site are located in the Kamienne Mts., a low mountain range in the Central Sudetes, SW Poland (Fig. 2). The study site in which the effect of landsliding was analysed is located at c. 800 m a.s.l., on the northern slopes on the Waligóra Mt massif (max 934 m a.s.l.), below the Suchawa peak (928 m a.s.l.). The topography of the study site is hummocky, typical of landslide slopes (Fig. 3A). Landslide relief and activity in the study area were described e.g. by Migoń et al. (2014) or Malik et al. (2016). The site is covered with a forest vegetation of Norway spruce (Picea abies Karst.) trees. The spruce trees growing on the study site have deformed growth habits. Their stems are tilted and bend along the slope inclination as a result of landsliding (Fig. 1A).
Fig. 3
A – Location of the Suchawa study site (L) and reference site (R). B – Location of the Hubertka study site (W) and reference site (R).
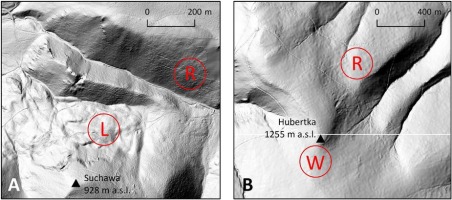
The reference site is located 0.4 km NE of the study site, on the SW slopes of the Krzywucha Mt massif, at c. 810 m a.s.l. The site is devoid of wind and landslide impact on tree growth. The reference site is shielded from wind by the surrounding mountain ridges and devoid of landslide relief (Fig. 3A). The forest cover of the reference site is identical to the forest growing in the study site. However, the trees which grow in the reference site have straight stems and undisturbed growth habits.
The Hubertka study and reference sites
The Hubertka study site and reference site are located in the Hrubý Jeseník Mts., the highest part of the Eastern Sudetes, NE Czech Republic (Fig. 2). The study site where the effect of the prevailing wind has been analysed is located at c. 1300 m a.s.l. The site is located on a slope, north of the flat top surface of the Praděd Mt massif (max 1491 m a.s.l.), above Hubertka peak (1255 m a.s.l.) (Fig. 3B). Westerly winds prevail in the area and the local topography of the study site increases this effect. The site is covered with a thin Norway spruce (Picea abies Karst.) forest of the upper tree line ecotone. As a result of the wind impact, the spruce trees growing in the study site are low. They have flagging crowns extending eastward and tilted stems (also in the eastern, leeward direction) (Fig. 1B).
The reference site is located 1.1 km NE of the study site, on the NE slopes of the Praděd Mt massif, c. 1100 m a.s.l. The site is devoid of wind and landslide impact on tree growth (Fig. 3B). It is shielded from wind by the surrounding mountain ridges and devoid of landslide relief. The Hubertka reference site is covered with a Norway spruce (Picea abies Karst.) forest of the upper montane vegetation belt. The trees have straight stems, normal heights, undisturbed growth habits and crown shapes.
. Study methods
We analysed tree-ring eccentricity among Norway spruce trees (Picea abies Karst.) in the study and reference sites (2 sites in the Kamienne Mts. and 2 in the Hrubý Jeseník Mts.) (Figs. 2, 3). We sampled 20 trees on the Suchawa landslide slope, 20 in the Hubertka study site affected by the prevailing wind and 10 trees in each reference site (in total: 20 reference trees not disturbed by landsliding and wind). In each study and reference site we selected trees visually assessed as healthy, devoid of visible losses in assimilation apparatus or stem injuries. Samples were taken using standard increment borers at breast height. Two cores were collected from each tree (120 cores in total). As regards trees growing in the Suchawa study site and Suchawa reference site, samples were collected along the axis parallel to slope inclination: one on the upslope and another on the downslope side of the stem (Fig. 1A). In respect of trees growing in the Hubertka study site, samples were collected along the west-east axis parallel to the prevailing wind: one on the windward and another on the leeward side of the stem (Fig. 1B). Sampling in the Hubertka reference site was performed similarly to the Hubertka study site, in the same west-east axis.
The cores were glued onto wooden holders and sanded to reveal the structure of tree rings. Tree-ring widths were measured with 0.01 mm accuracy (LinTab measuring station, TSAPWin Professional 4.65 software). The skeleton plot technique (Cropper, 1979) was used for cross-dating between the upslope and downslope or windward and leeward samples of individual trees to detect wedging rings and ensure the correct analysis and dating of eccentricity.
The ring widths were measured in all the samples. The measurement results for the opposite sides of individual stems were compared using the method of percent index of eccentricity. The eccentricity, eccentricity index and its yearly variation were calculated according to the formulas by Wistuba et al. (2013) (Fig. 4). The graphs of the index obtained for individual trees were analysed. We looked for any potential patterns such as e.g. long-term changes in the value of the eccentricity index over time. We compared statistical indicators of eccentricity (i.e. percent index) produced by trees in all four analysed sites: minimum values, maximum values, means, standard deviations and medians.
Fig. 4
Subsequent steps of tree-ring eccentricity analysis in a tree affected by landsliding in the Suchawa study site with respective formulas allowing transformation of ring widths [mm] on the upslope (Ux) and downslope (Dx) sides of stems into eccentricity [mm] (Ex), then eccentricity index [%] (Eix) and its yearly variation [percentage points] (vEix) and disturbance (eccentricity) events dated with the use of reference thresholds (method after Wistuba et al., 2013). Example of ring width graph, eccentricity index graph, graph of yearly variation of eccentricity index.
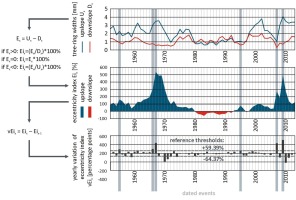
We also dated disturbance (eccentricity) events caused by landslide and wind activity on the study sites (Fig. 4). Data from stable, reference slopes were used in the dating procedure as thresholds. Reference thresholds were calculated separately for each study site, based on all values of the yearly variation of the index obtained for all annual rings in matching sets of 10 reference trees. The thresholds were calculated as a sum of averages and standard deviations for the sets of positive and negative variation values (index increases and decreases) separately (Wistuba et al., 2013). For example, the upslope reference threshold for Suchawa was calculated based on an average value of 29.11% and standard deviation of 30.28% which makes 59.39%, while the downslope reference threshold was calculated based on an average value of –30.98% and standard deviation of 33.39% which makes –64.37% (Fig. 4).
Disturbance events were dated in samples taken from the study sites as the years in which the values of index variation exceeded the thresholds (Fig. 4). Finally, we calculated the response index (Shroder, 1978): a percentage of trees with disturbance (eccentricity) events dated in a certain year in relation to the total number of sampled trees already alive in the same year (sample depth value) (Fig. 4). We analysed changes of the response index during periods when over 50% of the sampled trees were already alive (sample depth >50%) for both study sites.
. Results
Patterns of eccentricity developed by individual trees in the study sites and reference sites
Graphs of tree-ring eccentricity index were used to analyse patterns of eccentricity developed by individual trees in each study site and reference site (e.g. Figs. 4, 5). We determined the characteristic patterns of eccentricity among 20 trees sampled in each study site and 10 trees sampled in each reference site. We have found that eccentricity among trees affected by landsliding in the Suchawa study site (e.g. Figs. 4, 5) can be developed in both directions: upslope and downslope. The direction of eccentricity can switch abruptly with alternating longer periods of upslope and downslope eccentricity. The level of eccentricity index can reach up to hundreds or thousands per cent. Periods with constantly escalated levels of eccentricity index can last several years and alternate with periods of low eccentricity or no eccentricity (when the eccentricity index oscillates around 0%). During the life of one tree (decadal time scale), several periods of escalated eccentricity and several periods of low eccentricity can be usually identified in trees growing in the Suchawa study site (e.g. Fig. 4).
Fig. 5
Examples of tree-ring eccentricity developed by individual Norway spruce trees affected by landsliding (Suchawa study site), by prevailing wind (Hubertka study site) and by trees growing in reference sites, in the absence of landsliding and prevailing wind (Suchawa reference site and Hubertka reference site). Note the difference in vertical scale between the eccentricity index developed in the Hubertka study site and in other sites.
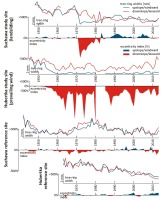
In the Hubertka study site affected by the prevailing wind, different patterns of eccentricity have been found (e.g. Fig. 5). Most of sample trees develop leeward eccentricity for the most of their lives. Single years with opposite, windward eccentricity can be found during the early years of tree growth, when eccentricity index values are low. After the first few years of tree growth, eccentricity becomes constantly leeward and its level escalates with age of each specimen. Once the level of eccentricity index escalates it rarely drops back to be weaker than – 100%. In general, the level of eccentricity index in trees growing in the Hubertka study site can reach up to hundreds or thousands per cent. However, extreme values occur only during short periods and do not last longer than 1–2 years (Fig. 5). During the life of one tree (tens to hundreds of years) several such events can usually be observed.
The patterns of eccentricity developed by single trees in both reference sites (the Suchawa reference site and the Hubertka reference site) (Fig. 5) are similar to one another. In all reference trees individual years or short periods with upslope and downslope or windward and leeward eccentricity occur alternately without any clearly visible trend. The values of eccentricity index found in trees from the reference sites oscillate around 0% most of the time (Fig. 5). They can exceed ±100% only in individual years.
Statistical parameters describing eccentricity developed by populations of trees from the study and reference sites
We have found clear differences between the statistical parameters calculated for the sets of eccentricity index values from the study sites and the reference sites (Table 1). The maximum values of eccentricity index found on the Suchawa and Hubertka study sites are distinctly higher compared to both reference sites. However, the maximum positive value in the Suchawa study site (affected by landsliding) is c. 3 times higher than in the Hubertka study site (affected by the prevailing wind). On the other hand, the minimum negative value of the index found in the Hubertka study site (influenced by wind) is not only significantly lower than the one in reference sites but also 10 times lower compared to the Suchawa study site (influenced by landslide activity) (Table 1). In general, the eccentricity index is more variable and gains more extreme values in sites affected by landsliding and the prevailing wind than in the reference sites. The same effect can clearly be observed in the mean values of the eccentricity index (both in general, and in separated sets of upslope/windward and downslope/leeward eccentricity) (Table 1). Higher variability of data sets from the study sites (in comparison to reference sites) clearly stems from the difference in the values of the standard deviation calculated for all four sites. Among the study sites, the standard deviation is higher in the Hubertka study site affected by the prevailing wind compared to the landslide slope of the Suchawa study site (Table 1).
Table 1
Statistical parameters describing sets of eccentricity index values calculated for sample populations of trees affected by landsliding (Suchawa study site), prevailing wind (Hubertka study site) and for sample populations of reference trees growing in sites unaffected by landsliding and prevailing wind (Suchawa reference site, Hubertka reference site). Upslope/windward (up./wind.) and downslope/leeward (down./lee.) eccentricity was separated in the calculations.
All statistical parameters calculated for the Suchawa and Hubertka study sites clearly indicate that, in the former case, upslope eccentricity is developed as a result of landsliding and that, in the latter case, leeward eccentricity is developed due to the prevailing wind. The median value for all tree rings from the Suchawa study site is slightly shifted above 0%, in the direction of positive values, while the median for the Hubertka study site is strongly negative, clearly shifted below 0%. At the same time, medians for whole data sets from reference slopes are close to 0% (Table 1). A similar tendency can be observed for mean values calculated for entire (upslope and downslope or windward and leeward) data sets (Table 1). The extreme value of leeward eccentricity developed in the Hubertka study site is an order of magnitude greater compared to the extreme value of windward eccentricity. The same can be observed for Suchawa, however, with upslope eccentricity prevailing (Table 1). There is also a clear disproportion between annual rings with opposite directions of eccentricity. In the Suchawa study site upslope eccentricity predominates (68.85% of the annual rings analysed), in the Hubertka study site a significant dominance of leeward eccentricity can be observed (84.10% of the annual rings under analysis), while on both reference slopes the proportions are almost even (Table 1).
Events of eccentricity dated on the study sites
The events of eccentricity in both study sites were calculated based on the number of trees with a yearly variation of eccentricity index exceeding the reference thresholds (Fig. 6). The reference thresholds for Suchawa are: +59.39 percentage points and –64.37 percentage points (for increases and decreases of eccentricity index, respectively). For Hubertka these are: +57.19 percentage points and –50.06% percentage points (for increases and decreases of eccentricity index, respectively). In both study sites we analysed the temporal variability of the response index calculated based on the number of eccentricity events among the sample trees (Fig. 6). In each case, we analysed only the period when the sample depth already exceeded 50% (since 1934 in the case of the Suchawa study site, and since 1949 in the case of the Hubertka study site).
Fig. 6
Sample depth and the number of eccentricity events dated in all trees sampled in both study sites. The response index calculated for the Suchawa study site (landslide impact) and for the Hubertka study site (impact of prevailing wind) with periods of sample depth >50% marked.
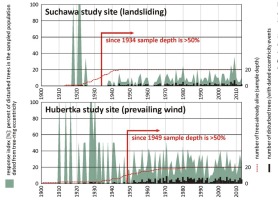
Between 1934 and 2013 the response index values exceeded 20% in the Suchawa study site in (Fig. 6): 1980, 2007 (25% each year) and 2009 (35%, a 1934–2013 maximum). Between 1949 and 2013 the response index values exceeded 20% in the Hubertka study site in (Fig. 6): 1955, 1962, 1969–70, 1973, 1975, 1978, 1983, 1988, 2009, 2013 (20–30% each year), 1957, 1963, 1974, 1981–82, 1984, 1987, 1989, 1992, 1997, 1999, 2006, 2012 (30–40% each year), 1952, 1956, 1960, 1965, 1977, 1980, 2000, 2004 (40–50% each year) and 1967 (50%, a 1949–2013 maximum). Therefore, the response index in the Hubertka study site not only gains higher values compared to the Suchawa study site, but also has a higher frequency of years with a response index over 20% (5.1 per 10 years on avg. compared to 0.38 per 10 years on avg.). From a long-term perspective, the response index pattern is more even in the Hubertka study site, where increased values of the response index occur in individual years, but frequently, and the response index level is almost evenly high during the whole period under discussion (Fig. 6). On the other hand, the extreme values of the response index in the Suchawa site are rare and are interrupted with long periods displaying a much lower level of this indicator (Fig. 6).
. Discussion
In the tree-ring record obtained from the landslide slope (the Suchawa study site) we found periods with an escalated level of eccentricity index in individual trees, which can be interpreted as periods of increased landslide activity, while periods with eccentricity index values oscillating close to 0% (the lack of tree-ring eccentricity and almost concentric growth) can be interpreted as periods when the slope under study was stable (inactive) (Figs. 4, 5) (e.g. Malik and Wistuba, 2012; Wistuba et al., 2013; Migoń et al., 2014; Łuszczyńska and Wistuba, 2015; Papciak et al., 2015). The same interpretation concerns also the increases and decreases in the response index from the entire landslide slope (Fig. 6), where we found brief periods of increased landslide activity separated by longer periods of stability. Therefore, the eccentricity record from the Suchawa study site reflects temporal variation in bedrock instability. The predominance of upslope eccentricity in the tree-ring record from the Suchawa study site (Table 1) is directly related to the nature of landslide movement. Rotational landslides, which are typical of the study area (Migoń et al., 2010), are characterised by concave sliding surfaces and by back-side tilting of the ground surface along with the trees which grow on the landslide blocks. According to Braam et al. (1987) and Wistuba et al. (2013) who described patterns of backward tree tilting on landslide slopes, upslope tilting is typical of upper and middle sections of rotational landslides, such as the study area (Fig. 3). Coniferous trees, if tilted upslope, tend to produce upslope eccentricity (Braam et al., 1987; Wistuba et al., 2013). Downslope tilting can, however, be found in the lowest and steepest part of the landslide toe and on the steep fronts of rotated landslide blocks (Wistuba et al., 2013). Therefore, the pattern of eccentricity in the Suchawa study site is not uniquely upslope (Table 1, Figs. 4, 5).
Trees from the Hubertka study site, which are affected by the prevailing wind, have a different eccentricity pattern compared to the above-described pattern produced due to landsliding. The general eccentricity of trees affected by the prevailing wind is leeward. This is due to tree stems being tilted along and with the direction of the westerly wind. It is also caused by the asymmetric growth habit of tree crowns which are flagging in line with the direction of the prevailing wind. Both features of the tree growth habit developed gradually, as branches on the windward side of a stem were eliminated (Schweingruber, 1996; Telewski, 2012). The windward branches were bent and broken by the wind. They could have been also broken as a result of overloading with snow or rime frost deposited on the windward side of tree crowns. Both crown flagging and wind impact itself can cause tree stems to tilt leeward and to develop leeward eccentricity as an expression of gradual adaptation of trees to the constant influence of the prevailing wind.
However, wood anatomy features such as: growth release, resin ducts, resin pockets, vessel distribution and reaction wood are the most often used in dendroecological studies on wind (e.g. Schweingruber, 1996; Duncker and Spiecker, 2008; Zielonka and Malcher, 2009). Eccentric growth is usually considered only in relation to cross-sections of whole stems (e.g. Robertson, 2011), but not in relation to individual annual rings. The connection between crown asymmetry in coniferous trees and the level of stem eccentricity was described by Burkhalter (vide Schweingruber, 1996). Tomczak et al. (2012) found stem eccentricity in pines to decrease with increasing distance from the edge of a forest exposed to strong winds. Schweingruber (1996) additionally indicated wind erosion of the cambium of Pinus longaeva on the windward sides of stems, which limited radial growth to only one, leeward side of the stems where live cambium is preserved.
As suggested by Robertson (1986), the eccentricity of single tree rings is strongly related to crown deformation and the mean direction of wind flow. The results from the Hubertka study site demonstrate that the eccentricity of single annual rings developed as a result of the prevailing wind escalates with time (and with the age of trees) (Fig. 5). This escalation can be related to the gradual adaptation of tree growth habits to the influence of wind, and is accumulated over time with the increasing age of trees. The impact may also increase over time because young trees are low and shielded from wind by the surrounding higher vegetation. Only after the height of a tree increases does it start to be exposed to the prevailing wind. In addition, the increase of tree age itself can result in an increase in eccentricity. When the tree ages, its size and weight increase. A greater weight increases the level of mechanical strains inside the tree stem, and these strains constitute a direct reason for the development of tree-ring eccentricity (Braam et al., 1987; Kojs et al., 2012).
Reference trees, growing in sites unaffected by landsliding and wind produce weaker ring eccentricity compared to trees affected by both those disturbing factors. In reference trees eccentricity index values oscillate around 0% and rarely exceed ±100%. These two characteristics, along with the frequent changes between upslope and downslope or windward and leeward eccentricity, are probably a record of a constant balancing of trees which struggle to maintain the near-vertical position and equilibrium on slopes. Balancing is an effect of the continuous need to fight the impact of disturbing factors as e.g. soil creep, snow cover and the increase in tree weight caused by tree growth over time (Gardiner et al., 2016).
The comparison of the records of tree-ring eccentricity typical of landslide activity and the prevailing wind (Table 1, Figs. 5, 6) clearly illustrates that the eccentricity developed in the Hubertka study site, influenced by wind, is stronger than that on the landslide slope of the Suchawa site. The difference in the level of growth disturbance is clear from the graphs of eccentricity developed for individual trees (Fig. 5), as well as from data collected for entire study sites: statistical parameters (Table 1) and response index graphs (Fig. 6). Therefore, the results indicate that the impact of the prevailing wind on the radial growth of trees is more severe than the one of landsliding. A significant impact of wind on ecosystems and trees in particular was emphasised by e.g. Ennos (1997) and Gardiner et al. (2016). Wind has profound consequences not only for the shape and size of stems and branches, but also roots (Gardiner et al., 2016). Plants exposed to high winds may also develop a limited number of smaller leaves (Telewski, 2012). The list of wind-related disturbances to plant anatomy and physiology includes: (i) a reduction in longitudinal stem growth, (ii) a stimulation of secondary radial stem growth and (iii) reallocation of biomass to the root system. Wind can even cause changes to the cell structure in both woody (e.g. Bannan and Bindra, 2011) and non-woody parts of plants (e.g. Gardiner et al., 2016).
The difference in the extent of landsliding and wind impact on tree growth may result from the study landslide being a slow-moving one (Malik et al., 2016). Slow-moving landslides can present rates of movement of less than a centimetre per year (e.g. Noferini et al., 2007; Massey et al., 2013) and their impact on tree growth can, therefore, be weaker compared to sudden, catastrophic landsliding. Previous studies show that the values of the response index calculated for landslide slopes in terms of tree-ring eccentricity can often exceed 40% (e.g. Wistuba et al., 2013). However, the frequency of such strong events on landslide slopes does not match the high frequency found in the Hubertka study site influenced by the prevailing wind. Therefore, wind impact should be carefully taken into account in dendrogeomorphic research as it can influence the results of landslide dating, as found e.g. by Martin and Germain (2015) for avalanches. These authors have found that growth disturbances caused by windthrows can be mistaken for the impact of snow avalanches. Particular caution should be exercised in respect of sites where signs of prevailing winds are present e.g. in the growth habit of tree crowns.
. Conclusions
The results show that patterns of eccentricity developed as a result of landslide activity and the prevailing wind differ from one another and from the eccentricity developed in the reference sites with no such disturbing factors. Differences have been found both in the patterns developed by individual specimens and in the patterns of the response index from entire sample populations of trees.
Norway spruce trees growing on an active landslide have a normal height of stems and growth habit of crowns. However, their stems are tilted along the general direction of slope. They develop both upslope and downslope eccentricity with the level of eccentricity index reaching up to hundreds or thousands per cent. The predominance of upslope eccentricity results from the cyclical character of the landslide under study. Prolonged periods with an escalated level of eccentricity index alternate with periods of low eccentricity or no eccentricity. The pattern records the temporally variable activity of the landslide on which the trees grow.
Spruce trees influenced by the prevailing wind have shorter stems, asymmetric and flagging crowns, and stems tilted leeward. At the beginning of their growth they develop weak windward and leeward eccentricity. When trees age, the eccentricity index escalates to hundreds or thousands per cent (with extreme values lasting up to 2 years) and the eccentricity becomes constantly leeward. The pattern records the adaptation of trees to the prevailing wind as the stems are tilted and branches on the windward side of a stem are eliminated and a flagging growth habit develops.
Spruce trees not subjected to the impact of landslide activity and prevailing winds have straight stems and undisturbed crown growth habits. They are characterised by the values of eccentricity index of around 0% and an alternating occurrence of single years or short periods with upslope and downslope or windward and leeward eccentricity. The pattern probably records constant balancing of trees maintaining a near-vertical position on slopes.
All the statistical parameters of eccentricity suggest that the impact of the prevailing wind on tree growth is more severe than the impact of landslide activity. The severity of wind impact on tree growth indicates that it should be carefully taken into account in dendrogeomorphic research as it can influence the results of dating. On the other hand, the possible influence of mass movements should also be considered in dendroecological studies of wind impact on trees.